Reports: DNI655184-DNI6: Visualizing Molecular Organization and Energy Transport Dynamics at Organic Surfaces and Heterojunctions with Interface Specific Femtosecond Spectroscopy
Sean T. Roberts, PhD, University of Texas at Austin
Organic semiconductors (OSCs) are a unique class of petroleum-derived materials that blend the processing advantages of plastics with the electrical properties of semiconductors. In contrast to inorganic semiconductors such as silicon and GaAs, OSCs can be readily processed from solution into highly-absorbing, thin, conductive films, making OSCs attractive materials for a wide range of optoelectronic applications. In many of these applications, the transfer of charge to and from OSCs is fundamental to device operation. The primary goal of our work is to develop a series of interface-specific, nonlinear spectroscopies that can be used to investigate OSC material interfaces to establish how the molecular organization of these regions controls their ability to donate and accept charge. During the past calendar year, we have achieved two key milestones for this project that are described in detail below:
Develop ESFG into a Tool for Interrogating the Electronic Structure of Buried OSC Interfaces
Electronic Sum Frequency Generation (ESFG) is a nonlinear process wherein two photons mix within a sample to generate a third photon at their sum frequency. Due to cancellation of forward and backscattering waves, ESFG is preferentially emitted from sample regions that lack inversion symmetry. As buried OSC interfaces naturally lack such symmetry, ESFG can selectively probe the electronic structure of these regions. OSC-based devices commonly contain multiple material layers, making it necessary to develop a model that can extract information regarding a specific interface of interest from ESFG spectra of OSC sample films. During our first year of support, we focused our efforts on (1) the construction of an ESFG spectrometer and (2) the implementation of an optical model to decompose these spectra into contributions from each interface within a sample film. In February, we published a report in J. Phys. Chem. Lett. summarizing these efforts. Specifically, this report described ESFG spectra measured for copper phthalocyanine (CuPc) thin films deposited on SiO2 and used a transfer matrix-based model to extract the second-order susceptibility, c(2), of molecules at the buried CuPc:SiO2 interface. This quantity is analogous to the linear absorption spectrum of molecules at the CuPc:SiO2 interface, but also contains information related to the average orientation of molecules at this interface. By modeling the polarization dependence of the ESFG spectra, we found CuPc molecules preferentially align normal to the SiO2 surface, in accord with x-ray diffraction experiments.
We have subsequently measured ESFG spectra of perylenediimide (PDI) thin films. PDIs possess the potential to undergo singlet exciton fission (SF), a process wherein an excited electron in a spin-singlet state shares a portion of its energy with an electron on a neighboring molecule, creating a pair of spin-triplet excitons. As SF generates two excited electrons from a single photon absorption event, it offers a path towards achieving optoelectronic devices that rely on carrier multiplication. In October, we published a report in J. Phys. Chem. Lett. that characterized the rate and yield of SF in the PDI derivative N-N'-Dioctyl-3,4,9,10-perylenedicarboximide (C8-PDI). Interestingly, ESFG spectra of C8-PDI films deposited on SiO2 reveal that C8-PDI molecules at the SiO2 interface possess a narrower bandgap than molecules in the films bulk (Figure 1B). We attribute this shift to a change in the intermolecular packing of C8-PDI molecules at this buried surface due to a relaxation of crystalline strain. If this structural relaxation leads to a similar reduction in the energy of C8-PDI triplet excitations, it may prove beneficial to SF-based light harvesting systems as it can provide an energetic driving force for triplet exciton migration to PDI interfaces.
|
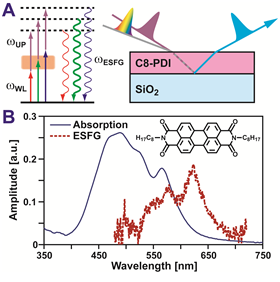
Figure 1: (A) In an ESFG measurement a white light continuum (rainbow) mixes with a spectrally narrow upconversion pulse (purple) in a sample, generating ESFG (light blue). Resonance between the sample and components of the WL enhances this mixing (left), allowing ESFG to serve as an interfacial analogue of linear absorption. (B) Linear absorption (blue) and ESFG reflection spectra (red dashed) of an 80-nm C8-PDI film.
Implementation of Heterodyne Detection for Electronic Sum Frequency Generation