Reports: ND554200-ND5: Advancing Radioactive Material Design by Understanding Nanoscale Nuclear Decay Effects in 2-D Films
E. Charles H. Sykes, Tufts University
The petroleum industry relies heavily on radioisotope probes at several stages of production including discovery of new reserves, monitoring oil flows, and quantifying the raw product as it emerges from the ground. Furthermore, oil and gas formations contain naturally occurring radionuclides that can be brought to the surface. Therefore, understanding how radionuclides interact with their local molecular environment and how they assemble in 2-D will produce novel methods for constructing nanoscale materials and technologies that will, in the long term, enhance the petroleum industry’s ability to use radionuclides for efficient production. 2D radioactive 125I monolayers are a recent development that combine the fields of radiochemistry and nanoscience. These Au supported monolayers show great promise for understanding the local interaction of radiation with 2D molecular layers (Figure 1), offer new directions for surface patterning, and enhance the emission of chemically and biologically relevant low energy electrons. However, the elemental composition of these monolayers is in constant flux due to the nuclear transmutation of 125I to 125Te and their precise composition and stability under ambient conditions has yet to be elucidated.
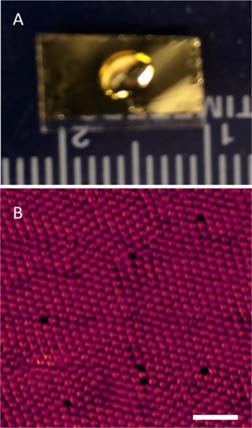
Figure 1 (A) Photograph of the deposition of 125I on Au(111) using an ambient dropcasting method (B) The resulting 125I monolayer as imaged using scanning tunneling microscopy (V = -0.4 V, I = 0.1 nA, scale bar = 2 nm).
The elemental composition of these monolayers is in constant flux due to the nuclear transmutation of 125I to 125Te, which occurs with a half-life of 60 days. Unlike I, which is stable and unreactive when bound to Au, the newly formed Te atoms would be expected to be more reactive. We have used electron emission and X-ray photoelectron spectroscopies (XPS) to quantify the emitted electron energies, track the film composition in vacuum and the effect of exposure to ambient conditions (Figure 2).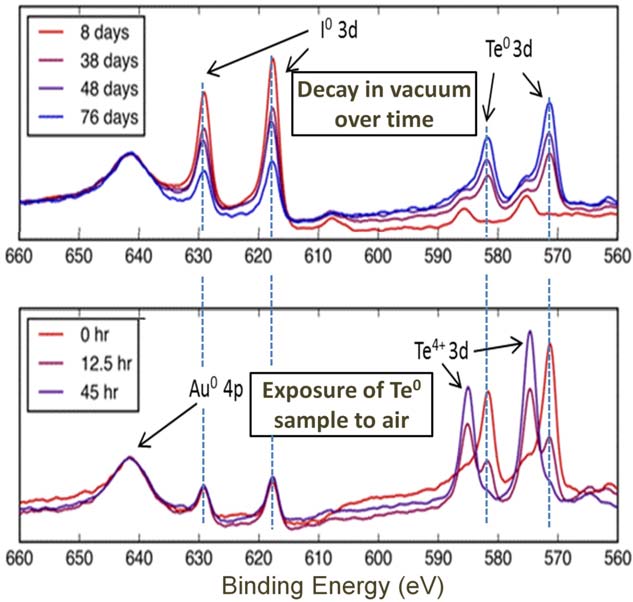
Figure 2 XPS spectra showing nuclear transmutation of I to Te and the effect of exposure to air. The upper plot shows the I 3d core levels decreasing and the Te0+ core levels increasing over time as the 125I decays into 125Te by electron capture. The bottom plot shows the formation of Te4+ caused by exposing a surface of Te0 formed in vacuum to ambient conditions for a number of hours.
XPS spectra indicate that the Te initially formed in its zero oxidation state upon exposure to air becomes oxidized to TeO2, which density functional theory (DFT) calculations confirm is exothermic by ca. 1.2 eV. Scanning tunneling microscopy (STM) allows us to visualize the surface composition and DFT simulated STM images enable assignment of both newly formed Te atoms as well as TeO2. Interestingly, we observe dimerization of the TeO2 into Te2O4 units, which DFT indicates are ca. 80 meV more stable than isolated TeO2. The Te in the I layer serves as a marker that allows us to demonstrate the facile mobility of the I films with time-lapse STM imaging. The fact that the Te2O4 units stay intact during major lateral rearrangement of the monolayer illustrates their stability (Figure 3). These results provide an atomic-scale picture of the composition and mobility of surface species in a radioactive monolayer as well as an understanding of the stability of the films under ambient conditions, which is a critical aspect in their future applications.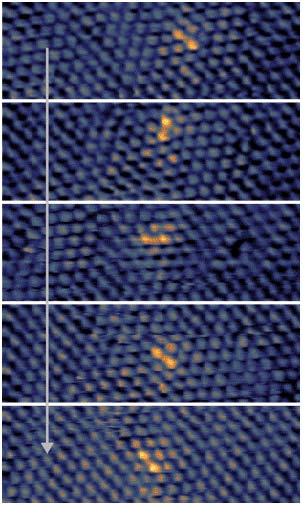
Figure 3 Diffusion of TeO2 dimer through the I monolayer. Shown is a series of consecutive STM images taken over a constant location.
In Figure 4 we observe the Te MNN Auger electron emission peaks at kinetic energies of 483 and 493 eV, which is at the same energy as a neutral Te atom.47 Based on literature photoemission data, one can expect that the Te MNN Auger peak will shift by around -1 eV (in kinetic energy) for each additional +1 charge on the Te atom.47 Monte Carlo studies of the decay of 125I atoms have shown that, on average, ~13 electrons are ejected per decay event33. Therefore, if the 125Te were charging up to +13 after being formed from 125I, the Te MNN Auger peak would appear drastically shifted to around 470 eV. However, it is clear from the data in Figure 2 that despite the ultra-fast electron emission cascades following a 125I EC event, the daughter Te atom does not charge up. In addition to the two predominant Te0 MNN Auger electron emission peaks we observe a smaller pair of peaks at 503 and 514 eV corresponding to I MNN Auger electron emission peaks. This would infer that some of the surrounding 125I atoms that have not yet decayed are excited by radiation in the form of either photons or electrons emitted during the radioactive decay.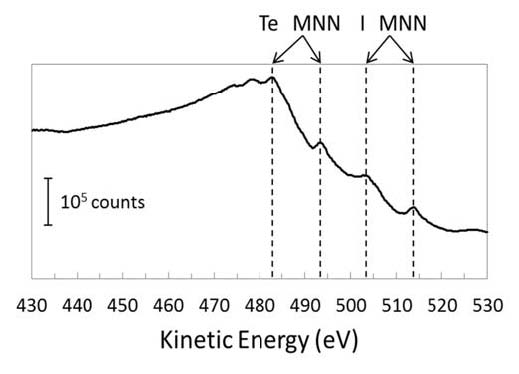
Figure 4 Kinetic energy spectrum of electron emission during radioactive decay of 125I on Au(111). Te MNN and I MNN Auger electron emission peaks are marked.
Overall, these results look very promising in terms of future applications of 125I/Au devices as both the radionuclide and daughter products remain strongly bound to the Au surface during both nuclear decay and exposure to ambient conditions. The reduced dimensionality of these surface-bound films provides the ability to study high energy nuclear processes safely and enables the microscopic details of radiation chemistry, biological degradation and material damage to be quantified. We envisage a new field that combines nanoscience with radioisotopes enabling understanding of many aspects of radiochemistry, physics and biology, as well as offering new constructs for in vivo radioisotope delivery for cancer therapy. Impact: This ACS PRF ND grant allowed the Sykes group to move in a different direction and investigate the nanoscale effects of radioactive decay in novel 2-D films. This fundamental work will enable the next generation of 2-D and nanoscale radioactive sensors, tracers and devices. This is a completely new area which the Sykes Group had no previous funding for.