Back to Table of Contents
44247-AC7
Complementary Hydrogen Bonding in Highly Branched Macromolecules: Thermoreversible Supramolecular Architecture for Improved Melt Processibility and Performance
Timothy E. Long, Virginia Polytechnic Institute and State University
Rationale:
Our
PRF-sponsored research program was focused on the potential synergy of tunable
intermolecular interactions and branched topologies as schematically
illustrated in the adjacent figure
(Figure 1.), and we uniquely correlated
novel macromolecular structure with application-specific physical properties
and performance for emerging technologies. Tailored intermolecular
interactions, including novel cationic polyelectrolytes and nucleobase hydrogen
bonding, will dramatically influence the performance of smart or self-healing
coatings and the transport of anions in alkaline fuel cell membranes for alternate
energy technologies. Moreover, our
research program identified a new family of ion-containing polymers that
combined nucleobase hydrogen bonding and associated pendant organic cations for
the formation of reversible, ion-channeled, macromolecular architectures.
Technological
Impact: Fatigued
polymers often contain micro-cracks and surface imperfections, which reduce
mechanical properties, optical performance, gas barrier properties, and tensile
performance. Self-healing polymers will extend the lifetimes of materials in
emerging technologies and minimize costly repairs and environmental impact.
Traditional healing methods for polymeric materials have included thermal
treatments with reversible organic structure, solvent processing, and imbedding
microencapsulated healing agents in polymer matrices. We identified
the design of novel functional polymers containing supramolecular interactions,
which are amenable to healing at mild conditions. Moreover, the potential
synergy of a branched topology with tailored intermolecular interactions offers
interesting promise for enhanced mechanical properties while maintaining
desirable rheological attributes such as shear thinning. Our research efforts
also involved the discovery of novel phosphonium cation containing block
copolymers for fuel cell membranes. Alkaline fuel cells (AFCs), which are often
based on a hydrogen fuel source in combination with a liquid electrolyte such
as aqueous potassium hydroxide, offer potentially superior performance compared
to other fuel cell systems operating below 200 oC.
Moreover, AFCs provide a more affordable
energy solution due to the suitability of less expensive non-noble metal
catalysts such as nickel and silver relative to platinum for sulfonated proton
exchange membranes. Future energy security demands our design of future
generations of alkaline anion exchange membranes (AAEMs) with improved
resistance to bicarbonate/carbonate formation, enhanced thermal stability for
AFC operation at elevated temperatures, increased resistance to hydroxide
degradation, and higher membrane conductivities.
Research
Milestones: The
research program was catalyzed from the preparation of a relatively unexplored
hydrocarbon-derived monomer containing a pendant trioctylphosphonium cation,
which was amenable to controlled free radical polymerization methodologies
using our novel difunctional bis-nitroxide initiator. In addition, collaborations
with Eastman Chemical and Kraton Polymers revealed the suitability of this
novel functional hydrocarbon platform for both adhesive and elastomer
technologies, respectively. The adjacent figure (Figure 2.) depicts the
building blocks for the introduction of nucleobase and phosphonium cation
functionality, and the implications on self-healing and alkaline anion exchange
membrane technologies were unprecedented. Ionomers possess desirable mechanical
properties and commercially viable products, such as chemically resistant
thermoplastics, coatings, and selectively permeable ion-transport membranes
have received significant attention. The figure below (Figure 3.) depicts the
thermomechanical performance of a phosphonium cation-containing block copolymer
with external phosphonium sequences with an internal acrylic block, and the
formation of nanoscale structure is evident from the formation of an extended
rubbery plateau region. Hydrogen
bonding in polymer structure also exhibits biologically relevant performance,
which offers significant potential as biomaterials, including drug and gene
delivery systems and biosensors. The physical cross-links that are derived from
microphase separation contribute to self-healing as well as mechanical integrity.
For hydrogen bonds, the strength and reversibility is highly dependent on
environmental conditions, such as temperature, solvent, humidity, and pH, but
the dynamic equilibrium for use in the application of self-healing has not been
addressed to a great extent in the literature.
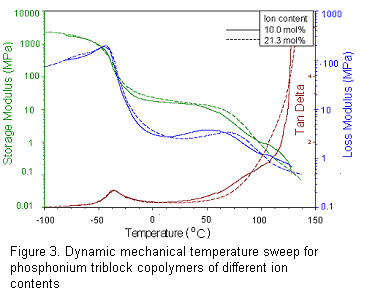
The research program has
specifically introduced nucleobases for complementary hydrogen bonding sites
(adenine and thymine) and phosphonium groups to polymers in order to
achieve repeated healing at mild conditions. The self-assembly of adenine- and
thymine-containing polymers serves to mimic the dynamic nature of nucleic
acids. Nucleobase-containing linear and branched copolymers exhibited DNA-like
melting behavior and a stronger temperature dependence of melt viscosity compared
to non-hydrogen-bonding polymers, suggesting possible advantages in
self-healing at relatively low temperatures.
Current studies involve a
comparison between phosphonium cation containing star copolymers and the
hydrogen bonding complexes of adenine block copolymers and uracil phosphonium
salts. In addition, the role of microphase separation in establishing hydrogen
bonding elastomers will also be studied by comparing morphological,
rheological, and mechanical properties of hydrogen bonding random copolymers
with those of block copolymers. In conclusion, this research program has
identified novel ion-containing monomers and hydrogen bond containing styrenic
monomers for conventional and controlled free radical polymerization. Theses
monomers have permitted the elucidation of intermolecular interactions on
mechanical properties with special attention on self-healing and anion
transport phenomena.
Back to top