Back to Table of Contents
44879-AC5
Direct and In Situ Investigation of Molecular Relaxation and Transport Properties of Nanocomposite Polymer Materials
René M. Overney, University of Washington
Of particular interest to the chemical processing industry
are reverse-selective membranes (also referred to as solubility-selective or
vapor-selective membranes). These membranes find
numerous chemical and refining industry applications including removal of
higher hydrocarbons from methane, olefin separation from nitrogen, and removal
of hydrogen from refinery process gas streams. In contrast to “conventional”
membranes, where diffusivities dominate the permeability, in reverse selective
polymers, the solubility ratio is the dominant factor, based on which larger
organic molecules can be separated from smaller permanent gases. Included in
this group of polymers is poly[l-(trimethylsily1)-1-propyne]
(PTMSP) and its analogues. These polymers have been shown to exhibit high
reverse-selectivity for organic vapor/permanent gas (e.g. n-butane/N2)
mixtures, and high glass transition temperatures, amorphous structures, and
extremely high free volumes.
The structures of PTMSP, as depicted in Fig. 1, show that the
polymer backbone contains rigid carbon-carbon double bonds. For PTMSP, bulky trimethylsilyl and methyl constituent groups are also
present, which result in very twisted molecules. The twisted conformation in
PTMSP is maintained due to the high energetic barriers (40
kcal/mol) to rotation around a backbone single bond. This creates an
extremely rigid chain that will maintain conformation achieved during
polymerization while changes in chain contour may still occur due to
fluctuations in dihedral angles in narrow potential energy wells.
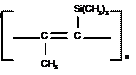
Fig. 1:
Structures of PTMSP.By incorporating fumed silica nanoparticles into the reverse
selective membranes, it was found that the permeability and diffusivity of the
material can be increased. Increases in free volume are thought as origin for
improved permeability. When fumed silica nanoparticles are incorporated into
the polymer matrix the rigid chains are unable to efficiently pack around the
particles. The morphology of the interfacial region is critical for determining
overall transport properties. With this project we have been investigating the
interfacial properties of polymer membranes and their effect on local gas
transport properties. In regards of PTMSP, we reported a weak binding interface
between fumed silica (FS) nanoparticle and the polymer matrix using a
heated-tip atomic force microscope (HT-AFM), with which we could peel off at
critical elevated temperatures near surface pinned nanoparticles.1
The HT-AFM methodology was specifically designed for this project (Fig.
2).
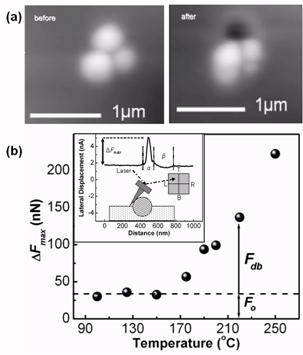
Fig. 2: (a) PTMSP-FS nanocomposite images before and after
a HT-AFM tip temperature of 250 °C. (b)
Lateral impact forc ΔFmax (see inset) as
function of temperature. Debonding occurred above 200 oC.
Fo
and Fdb
indicate the base force and debonding force, respectively.1
To illuminate the interfacial mobility properties of PTMSP,
we employed an in-house developed nanoscopic technique, Intrinsic Friction Analysis (IFA), to determine activated
relaxation process.2 With IFA, we identified the trimethylsilyl (TMS) side-chain rotation around the C-Si bond with 6.6 ± 0.5 kcal/mol, Fig. 3, as the
only activated relaxation process for film-thicknesses of
30 - 300 nm. This study revealed thickness-independent
activation energies within the margin of error. Thus, interfacial constraints
do not affect activated relaxation processes in PTMSP.
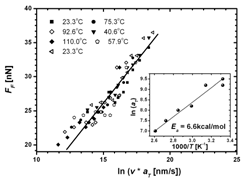
Fig. 3: IFA of PTMSP below 110 oC reveals an activated relaxation process of 6.6 kcal/mol that is identified as TMS side-chain mobility.
Based on recent findings involving macromolecular (backbone) mobilities
in polymers3 that affect the entropic component of “local”
submolecular mobilities in the interfacial region2, we concluded
changes in backbone fluctuations of PTMSP to be small compared
to the bulk. Thus, constrained backbone fluctuations cannot account for
the transport property enhancement in PTMSP-FS. This leaves (i) PTMSP particle-wettability, and (ii) polymer
free-surface predominant chemical groups that are present in the voids at the
interface, as the leading two causes for transport enhancement. While
poor wettability originates from stiff backbones, the bulk deviating chemical
environment in the void region is caused by the orientational
preponderance of side-chains towards the free surface2.
In ongoing research, we achieved first local reverse
selective flux results on PTMSP with flux lateral force microscopy (F‑LFM),
Fig. 4 and 5, which will be employed to explore transport variations in the
interfacial region of PTMSP-FS.
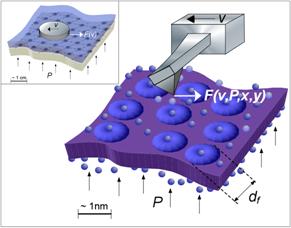 Fig. 4: (Left) Conceptual cartoon of F-LFM. Friction force, F, signals provide a measure of the fluid permeability, in analogy to the air hockey table (inset). (Right, top) Illustration of the instrumental setup. (Bottom) AFM flux stage.4 | 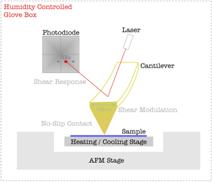 |
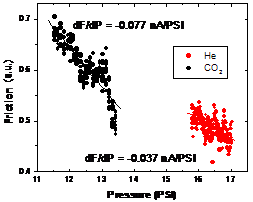
Fig. 5: Reverse selective transport of He and CO2 in PTMSP-FS (Left) Transient behavior measured macroscopically with a
bubble-meter. (Right)
Friction-pressure gradients (dF/dP) in F-LFM
measurements confirm the reverse selective behavior also on the local scale.
1 Killgore, J. P. and R. M. Overney,
Langmuir 24, 3446 (2008).
2 Knorr, D.B., T. Gray, and R. M. Overney, J. Chem. Phys. 129, 074504 (2008)
3 S. Sills, R. M. Overney, et al., J. Chem. Phys. 120, 5334 (2004).
4 J. H. Wei, M. Y.
He, and R. M. Overney, , J. Membrane Sci. 279, 608
(2006).
Back to top