Back to Table of Contents
45683-AC9
Using Colloidal Suspensions to Model Atomic Scale Lubrication Phenomena
Itai Cohen, Cornell University
As proposed, we have designed and built a new device
enabling simultaneous rheometry and visualization of colloidal suspensions in a
thin-film geometry. Our device is unique in its ability to realize a uniform
shear flow of extremely high aspect ratio, confining fractions of a milliliter
of sample between millimeter-sized parallel plates separated by as little as a
few microns. The entire device is loaded onto a confocal microscope allowing
direct observation of the suspension microstructure. Suspensions of
micron-sized particles have been used to explore a wide range of phenomena,
including nucleation,
melting, plastic flow,
and vitrification. In the present studies, we are exploring the effects of
confinement on the phase behavior and rheology of these suspensions.
Applying
oscillatory shear to a high volume fraction suspension, we have discovered
intermittent transitions between an elastic crystalline phase and a viscous
disordered fluid phase. This nonequilibrium behavior is highly unusual in a
number of ways. Previous studies on shear melting of colloidal crystals have
emphasized a smectic microstructure, consisting of particle layers sliding over
one another, which emerges through a shear banding phenomenon. In our
experiment, however, the smectic phase is not observed. As the applied strain
amplitude is increased sufficiently far, the suspension goes directly from
crystalline order to fluid-like disorder. Remarkably, the disordered phase
isn't stable either and the suspension later makes a sudden transition back to
its crystalline phase, which later destabilizes again. These intermittent phase
transitions are mirrored by dramatic fluctuations in our stress measurements,
as shown in Figure 1, where the large force spikes occurring when the
suspension is disordered.
In
the elastic regime, we use particle imaging velocimetry to extract oscillation
amplitudes at the upper and lower boundaries of the crystal. The induced strain
amplitude obtained from these measurements
is a factor of 30 smaller than the applied strain amplitude defined by the
relative motion of the upper and lower plates of the shear cell. Previous
experiments with less confined suspensions have observed a far smaller
difference between applied and induced strain, if any. This naturally raises
questions about the general importance of slip in confined suspension rheology
and, in particular, about its role in intermittent transitions between ordered
and disordered phases at higher applied strain amplitudes. Our working
hypothesis is that these transitions are part of a stick-slip phenomenon, in
which competition between self-organization principles and instabilities allows
the system to explore a range of induced strains. We are also considering
possible connections with other complex fluid phenomena such as rheochaos and
shear thickening.
We currently preparing to perform more in depth
measurements and analysis of both linear and nonlinear aspects of the system's
behavior as applied strain is varied, hoping to gain further insight into this
phenomenon. To further probe the role of
slip, we are designing experiments in which the boundary conditions are
altered. Cornell's microlithography facilities give us the ability to replace
the smooth surface morphology of our shearing plates with reproducible,
patterned boundaries that grip the colloidal particles. The development of this
process is nearly complete. We have already made numerous patterns and observed
that they can significantly reduce slip. The consequences of patterned
boundaries for colloidal phase and flow behavior is an important question in
general and should find tribological applications as well.
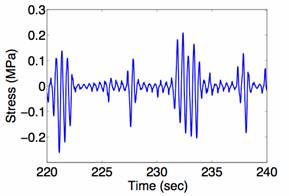
Figure 1. Intermittent stress
measurements
Back to top