Reports: ND456517-ND4: Dissipative Covalent Bond Formation for Non-Equilibrium Systems Chemistry
C. Scott Hartley, Miami University
As outlined in the original proposal, the project has three Specific Aims: (1) The development of anhydrides as dissipative covalent bonds. (2) The construction of macrocycles using this chemistry. (3) The generation of out-of-equilibrium molecular geometry changes using this chemistry. Over the past year, we have made substantial progress on Specific Aims 1 and 2 and have begun work on Specific Aim 3.
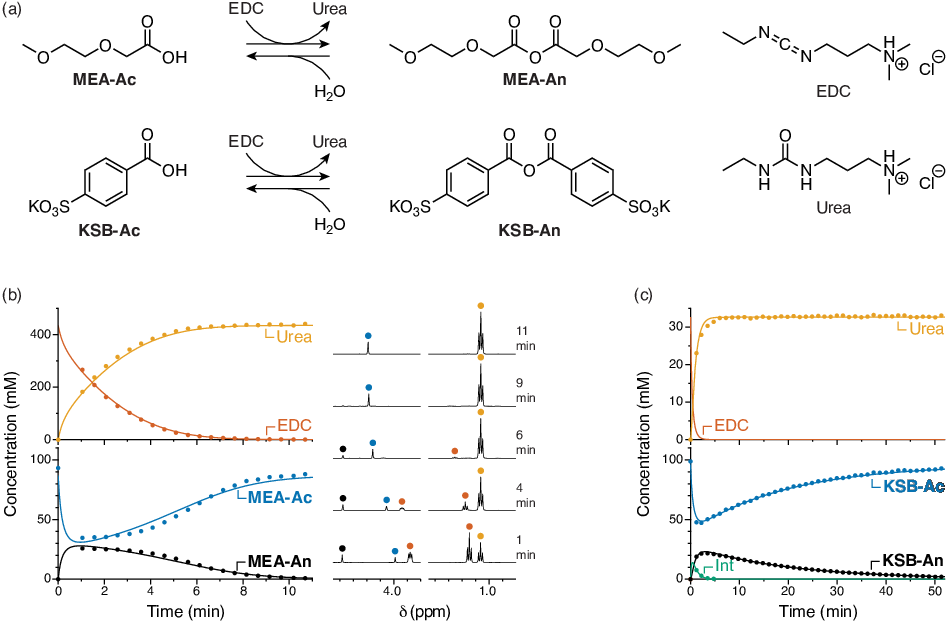
Figure 1. (a) Assembly of simple monoacids MEA-Ac and KSB-Ac into transient anhydrides, fueled by EDC. (b) 1H NMR monitoring of the MEA-Ac system. (c) 1H NMR monitoring of the KSB-Ac system.
Our first key results were to establish conditions for the transient assembly of simple commercially available carboxylic acids MEA-Ac and KSB-Ac fueled by the common carbodiimide reagent EDC, as shown in Figure 1a (Specific Aim 1). After some optimization, suitable conditions were identified and kinetic runs performed for both systems. Substantial characterization was done to show that anhydride is indeed formed in these systems. The kinetics of both systems are readily monitored by NMR spectroscopy (Figure 1b,c), and the concentrations of all species could be fit to a simple kinetic model (solid lines in the plots). From the fits we were able to extract further information about the systems, particularly the yields (net anhydride formed per EDC added).
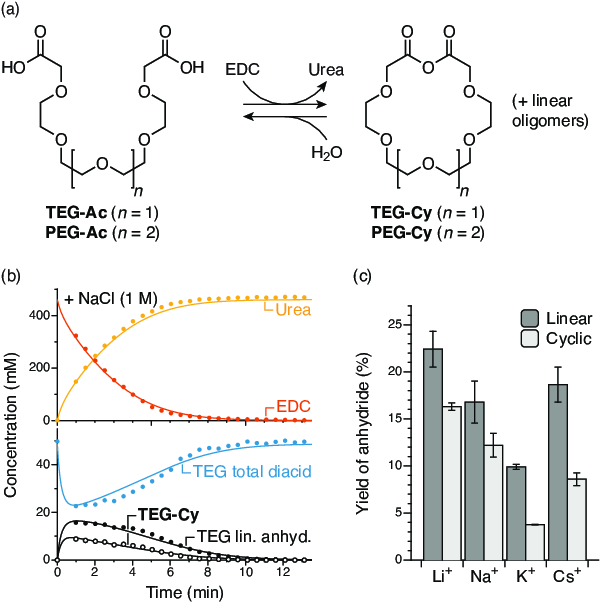
Figure 2. (a) Macrocyclization of diacids TEG-Ac and PEG-Ac fueled by EDC. (b) Typical kinetic run for the TEG-Ac system, in the presence of NaCl. (c) Yields of the macrocycle TEG-Cy and linear anhydrides in the presence of various cations.
These results demonstrate the basic feasibility of the system. To show that this chemistry could be used to assemble more complex architectures, we explored the formation of crown-ether-like macrocycles from simple diacids, as shown in Figure 2a (Specific Aim 2). The behavior of these systems is comparable to the monoacids, with both cyclic (TEG-Cy) and acyclic anhydrides observed (Figure 2b). Interestingly, the yields of the macrocycles were found to be sensitive to the presence of alkali metal cations, as shown in Figure 2c, with the “matched” cation acting to decrease the yield of the macrocycle. This effect, a sort of “negative templation” by the species’ own guest, is a counterintuitive example of out-of-equilibrium effects on assembly. We have published a paper describing these results in the Journal of the American Chemical Society.
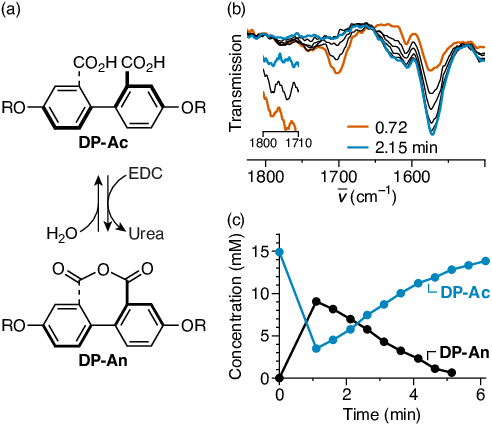
Figure 3. (a) Out-of-equilibrium anhydride formation in diphenic acids. (b) IR spectroscopy monitoring of the reaction of DP-Ac. The peaks at 1780 and 1740 cm−1 are indicative of the anhydride. (c) Kinetic profile for the reaction from 1H NMR monitoring.
We are presently continuing our efforts toward Specific Aims 1 and 2 by taking a careful look at structure–property effects in a systematic series of monoacids and by designing new cage-like hosts that should exhibit much stronger cation binding constants. Materials for these experiments are currently being synthesized. We have also made significant progress toward Specific Aim 3. We have shown that this same chemistry works on diphenic acids to give diphenic anhydrides (which results in a large decrease in the twist about the biaryl bond), as shown in Figure 3. We are currently synthesizing substituted diphenic acids to probe structure–property effects in this system.