Reports: ND453415-ND4: Mechanistic Studies of Transition-Metal Catalyzed, MAO-Assisted Olefin Polymerization
Rainer Ernst Glaser, Ph.D., MS, Dipl.-Chem., University of Missouri, Columbia
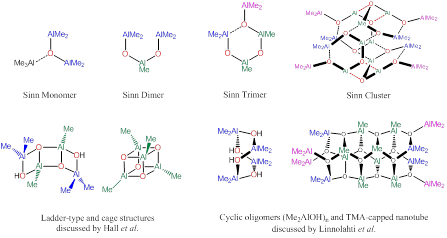
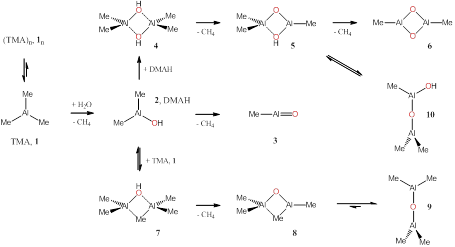
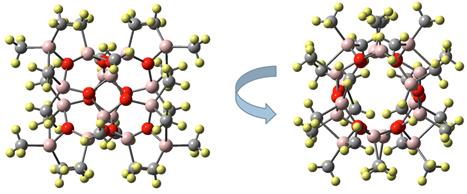
Rainer Ernst Glaser, Ph.D., MS, Dipl.-Chem., University of Missouri, Columbia
Copyright © American Chemical Society