Reports: DNI652494-DNI6: Dynamics and Kinetics of Carbon Dioxide in Nano-Porous Materials Environment from Quantum Molecular Dynamics Simulations
Yosuke Kanai, PhD, University of North Carolina (Chapel Hill)
|
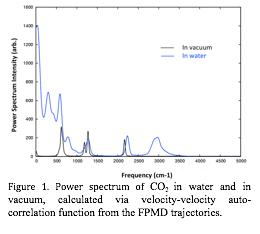
are appreciable (4−5) in water. Our
present study shows that long- range structural arrangements of water molecules
are also important to dictate the dynamical behavior of water molecules at
surfaces passivated by different hydrophobic
molecules. The observed structural dependence on the dynamics of interfacial
water is likely to be a universal character for a wide range of surfaces.
During the investigation of the CO2
hydrolysis to bicarbonate ion in bulk water, it has become clear that classical
molecular dynamics simulations are necessary to address some important
dynamical properties such as diffusion constant of the water molecule when CO2
molecule is solvated. First-principles molecular dynamics (FPMD) turns
out to be computationally too expensive for computing the diffusion constant
accurately in terms of statistical sampling. At the same time, most classical
molecular dynamics is known to suffer from the inadequate treatment of charge
transfer. Building on the recent work by Rick and co-workers (1), we
investigated the performance of the new potential that can take into account
both electronic polarization and charge transfer. Classical molecular
dynamics simulations were performed using three different force fields: a fixed
charge force field (SPCE/HMN), a polarizable force field that includes explicit
polarization (SWM4-NDP), and also a recently developed force field that
includes polarization and charge transfer (QF-DCT). These simulations were then
compared to FPMD simulations. We performed molecular dynamics simulations on
four types of systems containing ion and solvating water. Two systems contained
a cation (Na+