Reports: UR552145-UR5: Synthesis of Ruthenium Dioxide Nanoparticles and Clusters with Tailored Size-and Surface-Dependent Properties for Enhanced Catalytic Applications
Vladimir Kitaev, Wilfrid Laurier University
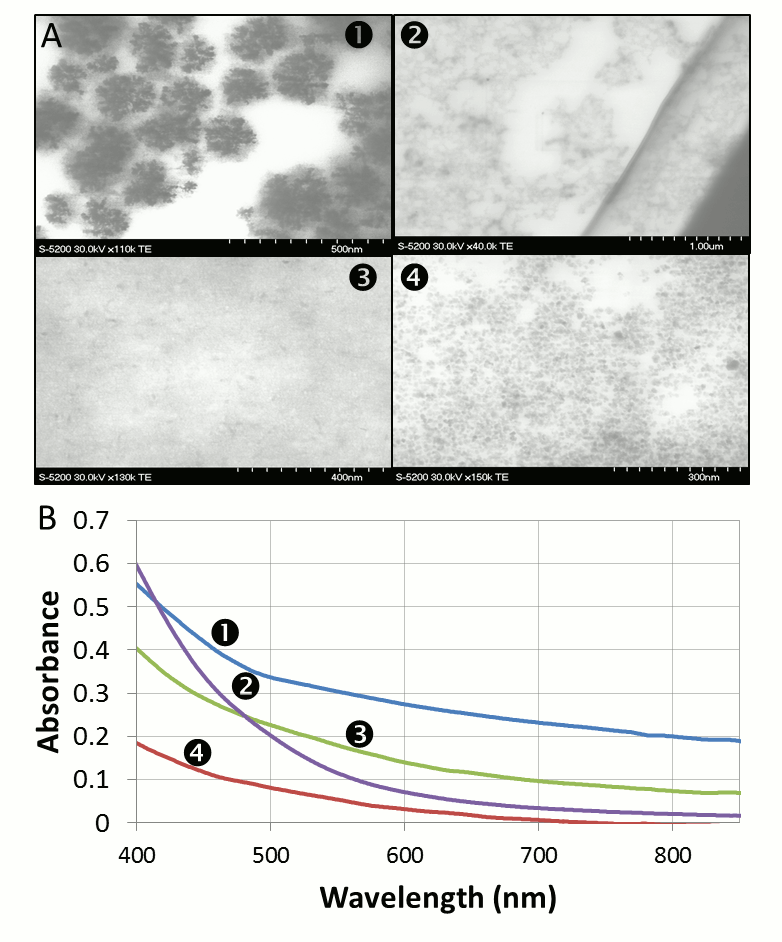
Vladimir Kitaev, Wilfrid Laurier University
Copyright © American Chemical Society