Reports: DNI553581-DNI5: Designer Graphene Nanostructures for Heterogeneous Catalysis
Enrico Rossi, PhD, College of William and Mary
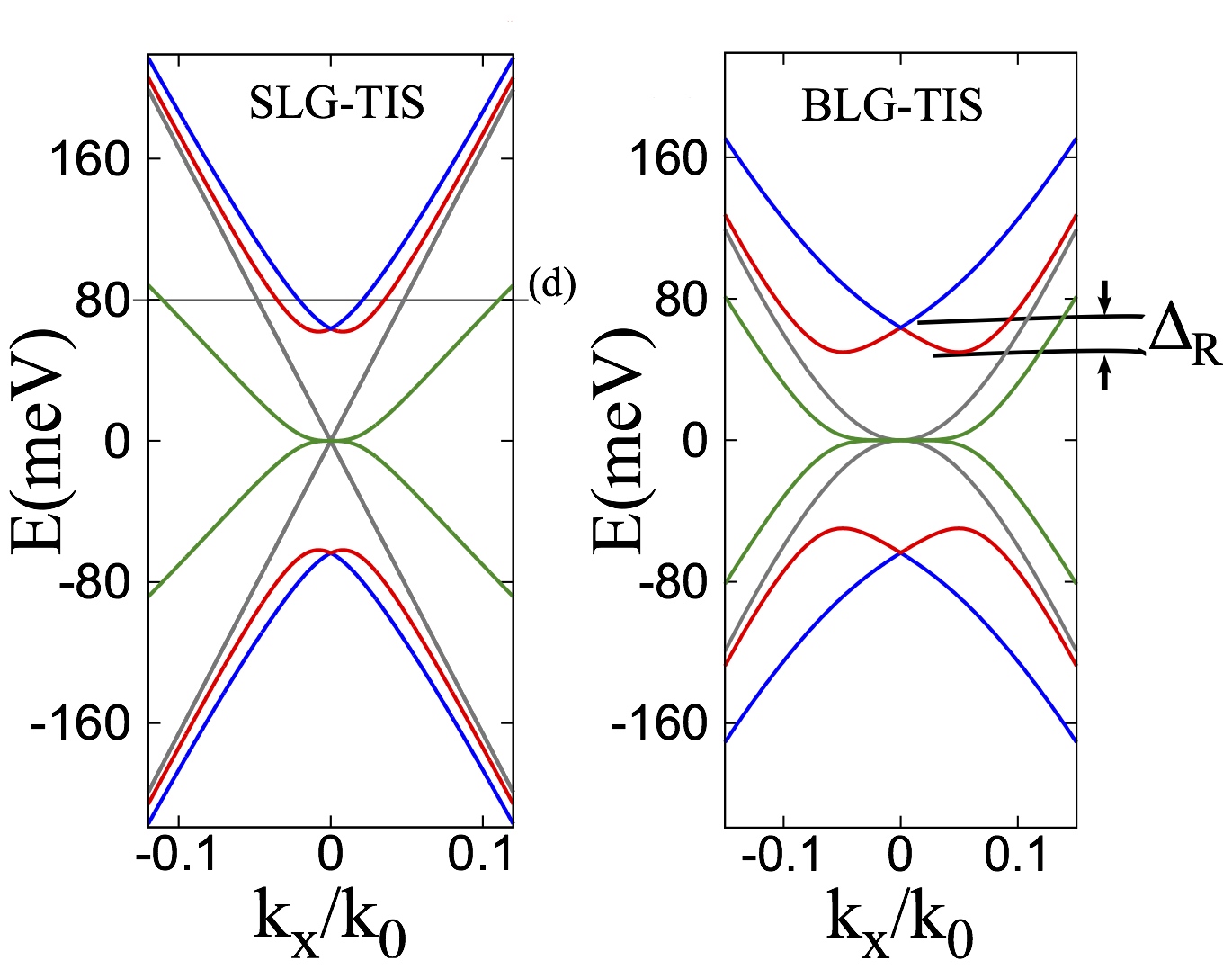
Figure 1. Electronic structure of commensurate SLG-TI and BLG-TI heterostructures. The grey line show the band structure of isolated SLG (BLG).
Figure
2. Example of spin structure on the Fermi surface of a TI-SLG
heterostructure.
Figure
3.
Sketch of a TI-Ferromagnet-Superconductor heterostructure.
Figure
4. Example of electron/hole puddles induced by disorder in a
graphene-graphene heterostucture. Panels (a) and (b) show the carrier density
profile in bottom, top, layer respectively. Panels (c), (d) show the profile of
the screened disorder potential.
Figure
5. Examples systems formed by graphene nanoribbons (red) placed on a sheet of graphene (blue).