Reports: UNI352868-UNI3: Alternative Clean Energy Production with Reusable Iron Catalysts: Development of Design Parameters for Future, Durable Green Energy Catalysts
Jesse W. Tye, PhD, Ball State University
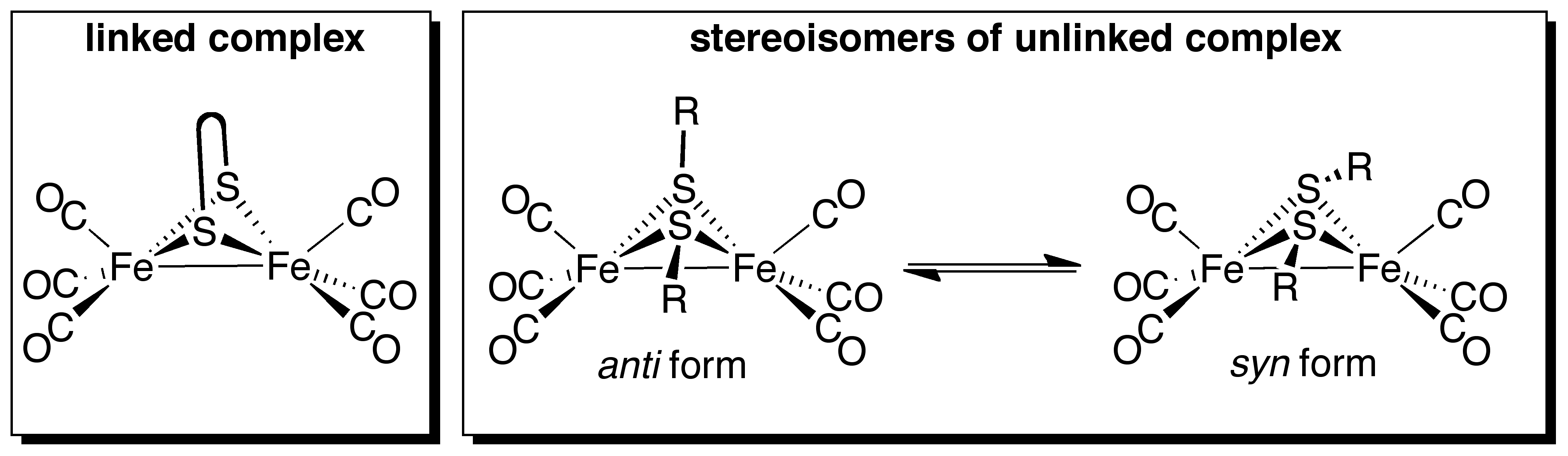
Jesse W. Tye, PhD, Ball State University
Copyright © American Chemical Society