Back to Table of Contents
44286-AC1
Bio-Inspired Cobalt Catalysts for Carbene and Nitrene Transfer Reactions
X. Peter Zhang, University of South Florida
1. Design
and Synthesis of New Chiral Porphyrins
New
members of chiral porphyrins ([H2(Por*)]) have been designed and
synthesized via palladium-mediated quadruple
amidation reactions of readily accessible bromoporphyrins with various chiral amide building blocks. Among this
family of D2-symmetric
[H2(Por*)], a group of six derivatives [H2(P1)]–[H2(P6)], possessing diverse electronic, steric, and chiral
environments, has been demonstrated as effective chiral ligands in supporting
Co-based carbene and nitrene transfer reactions. The structures of cobalt(II)
complexes of [H2(P1)]–[H2(P6)] are shown in Figure
1, along with those of two analogous achiral porphyrins [H2(P7)] and [H2(P8)].
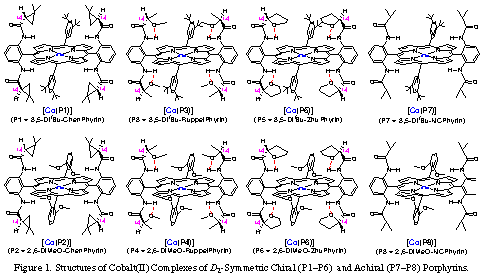
2.
Asymmetric Cyclopropanation of Alkenes
The
well-documented importance of cyclopropanes in numerous fundamental and
practical applications has stimulated vast efforts for the synthesis of the
smallest carbocycles. Metal-catalyzed asymmetric cyclopropanation of alkenes
with diazo reagents constitutes the most direct and general method for
stereoselective construction of the unique all-carbon triangular structures. A
number of outstanding chiral catalysts have been reported to achieve high
diastereo- and enantioselectivity for several classes of cyclopropanation
reactions, most of which employed styrene derivatives and some electron-rich
olefins with diazoacetates. Ongoing endeavors in the field aim at further
expansion of its substrate scope to include different types of alkenes and with
various kinds of carbene configurations.
2-1. Cobalt-Catalyzed Asymmetric
Cyclopropanation of Electron-Deficient Olefins
Asymmetric
cyclopropanation of electron-deficient olefins containing electron-withdrawing
groups such as a,b-unsaturated carbonyl
compounds and nitriles have proven to be a challenging problem presumably due
to the electrophilic nature of the metal-carbene intermediates in the catalytic
cycles. We have reported a general and efficient catalytic system for
asymmetric cyclopropanation of electron-deficient olefins. [Co(P1)] (Figure 1) was shown
to cyclopropanate a wide range of a,b-unsaturated carbonyl
compounds and nitriles (Table 1), forming the corresponding electrophilic
cyclopropane derivatives in high yields and selectivities. Furthermore, the
[Co(P1)]-based
catalytic process could be operated efficiently at room temperature
in a one-pot fashion with olefins as limiting regents and would not require the
slow-addition of diazo reagents.
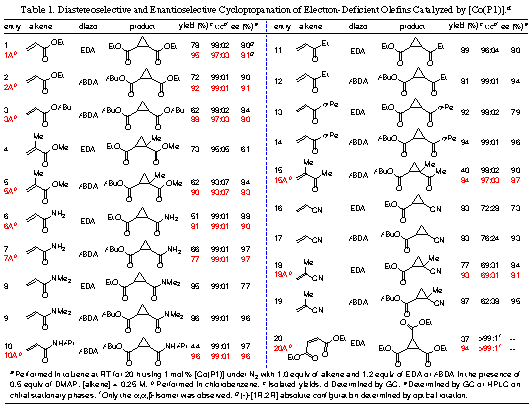
2-2. Cobalt-Catalyzed Asymmetric
Cyclopropanation with Diazosulfones
A
number of asymmetric catalytic processes have been successfully developed to
permit olefin cyclopropanation in high yields and high selectivities. While the
vast majority of those catalytic systems employed diazocarbonyls, mostly
diazoacetates, as carbene sources, metal-catalyzed asymmetric cyclopropanation
reactions with other types of diazo reagents are underdeveloped. To further
augment its substrate generality, we decided to explore the effectiveness of
the [Co(Por*)]-based catalytic system for asymmetric cyclopropanation with
diazo reagents, rather than diazoacetates. As a result of this effort, we have
reported that [Co(P6)] is a highly effective catalyst for asymmetric
cyclopropanation employing diazosulfones (Table 2).

3.
Selective Aziridination of Alkenes
Metal-catalyzed
olefin aziridination is a fundamentally and practically important chemical
process that has received increasing research attention. The resulting
aziridines, the smallest nitrogen-containing heterocyclic compounds, are key
elements in many biologically and pharmaceutically interesting compounds and
serve as a class of versatile synthons for preparation of functionalized
amines. In view of the similarity to diazo reagents for carbene transfer
processes, azides should have the potential to serve as a general class of
nitrene sources for metal-mediated nitrene transfer reactions, including
aziridination. In addition to their wide availability and ease of synthesis,
azide-based nitrene transfers would generate chemically stable and environmentally
benign nitrogen gas as the only by-product. Despite these attributes, only a
few catalytic systems have been developed that can effectively catalyze the
decomposition of azides for aziridination.
3-1. Cobalt-Catalyzed Effective Aziridination
with Arylsulfonyl Azides
Previously,
we reported that [Co(TPP)] can catalyze olefin aziridination with commercially
available diphenylphosphoryl azide (DPPA) as a convenient new nitrene source,
leading to the formation of N-phosphorylated aziridines. In an attempt to
expand the catalytic process for other azides, it was found that [Co(TPP)] was
ineffective for olefin aziridination with sulfonyl azides. As part of our
efforts to develop new porphyrin ligands to enhance Co-based catalytic
processes, we have reported the design and synthesis of a new porphyrin P7 (Figure 1) based on
potential hydrogen bonding interaction in the assumed metal-nitrene
intermediate. The Co(II) complex of P7 [Co(P7)] was shown to be a
highly active catalyst for aziridination of different aromatic olefins with
various arylsulfonyl azides, forming the corresponding aziridines in excellent
yields under mild conditions (Table 3).
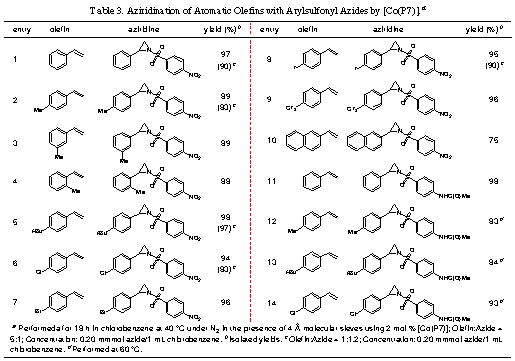
3-2. Cobalt-Catalyzed Asymmetric
Aziridination with Diphenylphosphoryl Azides
To
improve its catalytic efficiency as well as to develop its asymmetric variant,
we have made considerable efforts to identify chiral porphyrins to support the
Co/DPPA-based catalytic aziridination with hopes of enhancing its activity and
selectivity. Recently, we reported the results from our systematic studies
regarding the use of D2-symmetric chiral porphyrins (Figure 1) for the
Co-based asymmetric olefin aziridination using DPPA. In addition to improved
yields under milder conditions, acceptable asymmetric induction has been
achieved. This represents the first Co(II)-catalyzed asymmetric aziridination
process (Table 4).
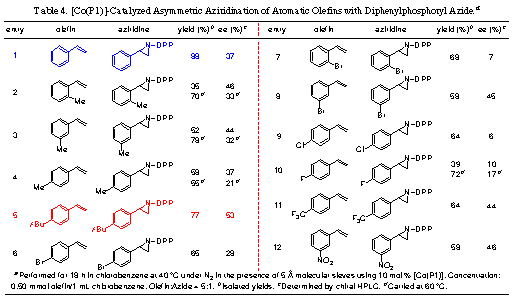