Back to Table of Contents
45995-G6
Real-Time Investigation of the Dynamic Structural Changes of Metal Oxide Nanocrystals using Time-Resolved X-ray Spectroscopy
Dong Hee Son, Texas A&M University
As a first step towards understanding the dynamic property
relationship in metal oxide nanocrystals, we focused on the two tasks: (1)
instrumental development for time-resolved structural study of nanocrystals
using short x-ray pulses and (2) initial time-resolved optical measurement of
the dynamic electronic and magnetic properties of iron oxide nanocrystals.
For the time-resolved study of the structural dynamics of
transition metal oxide nanocrystal in colloidal solutions, X-ray absorption
spectroscopy is an optimal tool. While the off-site synchrotron ultrafast X-ray
facility will be used initially and perhaps more often for the time-resolved
X-ray absorption measurements, a laser-based ultrafast X-ray source is also
being constructed in the laboratory. The laser-based X-ray source, which is a
part of the pre-existing laser system for the optical experiment, provides
practical convenience despite its weakness in intensity. So
far, we have built the X-ray source chamber and characterized the stability of
Hg jet-flow and recirculation inside a vacuum chamber and laser beam focusing
characteristics. Figure 1 shows a diagram of X-ray generation chamber
(top) and a picture of currently completed part of the X-ray generation chamber
(bottom). The vacuum chamber contains optics focusing the laser beam onto the
X-ray generation target, a circulation system for liquid mercury used as X-ray
generation target and an X-ray focusing optics. A stable jet of Hg (100 mm-thick) is formed using nitrogen gas as a
pressurizing medium, with only 5 minutes of interruption every ~2 hours for
recirculation. Laser beam size on Hg jet is ~15 mm
from an off-axis parabolic mirror, which will be further minimized to deliver
the highest intensity to Hg target.
Recently, a similar system operating in another group produced X-ray
pulses of 5×109 photon/4p×s within 1 keV width in 5-10 keV range,
using the laser system operating at 5 kHz and 15 W. Our initial target
performance will be in a similar range.
We
also investigated the electronic relaxation dynamics and demagnetization and
recovery dynamics in Fe3O4 nanocrystals, which can be
modulated due to optically induced coherent lattice motion. Pump-probe
transient absorption and Faraday rotation techniques, both with sub 100 fs
temporal resolution, were employed to obtain time-dependent electronic and
magnetic properties respectively. Figure 2 shows pump-probe transient
absorption and transient magnetization data of colloidal Fe3O4
nanocrystals of various sizes. At the pump wavelength of 780 nm, metal-metal
charge transfer transitions involving d-electrons of Fe ions were excited.
Electronic excitation also excited coherent acoustic phonon in the lattice and
also resulted in demagnetization via spin-orbit coupling. Modulation of transition
absorption by coherent lattice motion was clearly visible, while magnetization
was not noticeably affected. Another notable feature in Figure 2 is the
different size dependence of the electronic and magnetization dynamics. While the
former is not very sensitive to the size of nanocrystals, the latter exhibit
strong size dependence. Weaker sensitivity of the electronic relaxation
dynamics to the size may be explained in terms of the relatively localized
nature of d-electrons, which will experience weak spatial confinement. On the
other hand, magnetization dynamics clearly reveal stronger size dependence of
spin degrees of freedom. The data can be interpreted in terms of size-dependent
cooperativeness of spins, i.e. spin correlation: weaker spin correlation in
smaller nanocrystals results in more prompt and larger recovery of
magnetization. This is the first experimental result showing the size effect on
the dynamics of the magnetization induced by an ultrashort optical excitation
in transition metal oxide nanocrystals of <10 nm length scale.
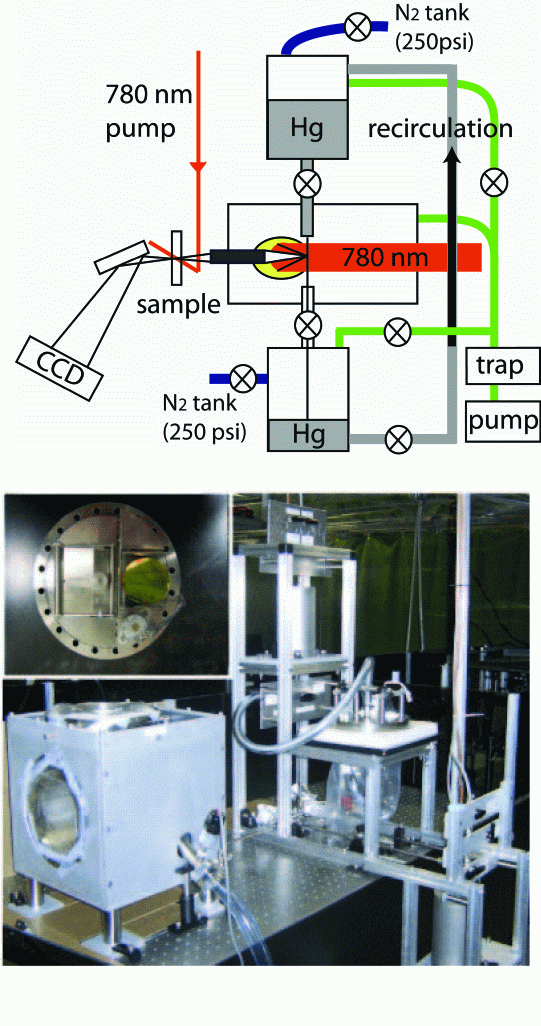
Figure 1.
Laser based X-ray source
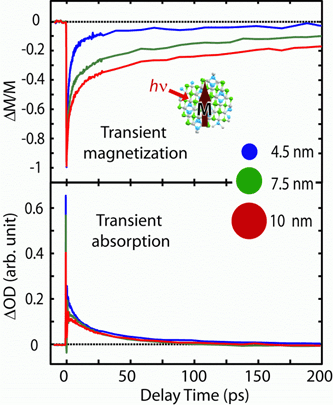
Figure
2. Size effect on transient
magnetization and transient
absorption
Back to top