Back to Table of Contents
47099-AC5
Hydrogenation Reactions on Palladium Involving Subsurface Hydride
Paul S. Weiss, Pennsylvania State University
����������� We have
been utilizing low-temperature, ultrahigh vacuum scanning tunneling microscopy
(STM) and spectroscopy (STS) to
examine the interactions of sulfur-containing heterocycles with a single
crystal palladium (Pd) surface.� We have
observed thiophene at low to moderate coverages on Pd{111} to ascertain its
adsorption orientation, low-temperature mobility, and electronic structure .� We are developing the methods necessary to
induce the reactions of individual molecules with pre-defined subsurface
hydride features.� These experiments will
help us elucidate the role of subsurface hydride in critical hydrogenation
processes reactions.
����������� We have
observed that both the surface preparation and the temperature at which
thiophene is deposited affects adsorption.�
Figure 1 shows STM
images representative of two separate dosing conditions, 4 K and ca. 20�40 K.� Dosing directly onto the surface at 4 K (Figure
1A) results in adsorption with random placement of molecules on the surface.� However, depositing immediately after the
crystal is transferred to the low-temperature chamber, we find that thiophene
adsorbs preferentially along the tops and bottoms of step edges, as seen in
Figure 1B.� In this case, thiophene
remains mobile while the surface cools, finding favorable binding sites along
step edges.
����������� We acquired
action spectroscopy over individual thiophene molecules to address their mobility
at 4 K.� Figure 2 shows A) a representative
action spectrum along with a sequence of STM
images acquired B) before, C) during, and D) after acquisition.� The molecule over which we chose to perform
action spectroscopy is denoted by the arrow in Figure 2A.� Because we acquire topography before and
after the spectroscopic point, we see behavior typical of a molecule that
becomes mobile beneath the probe (Figure 2B).�
That is, since the thiophene moves out of the tunneling junction during
spectroscopy and before topographic imaging is complete, we image only �half�
of the molecule.� Subsequent topographic
imaging shows that the thiophene indeed moved as a result of the tip
perturbation (Figure 2C, arrow).� From
these experiments, we are developing a technique that will allow us to
manipulate single molecules into proximity of subsurface hydride features patterned
in the substrate by STM manipulation.
����������� We have recorded
initial differential conductance spectroscopy (dI/dV) over both the
Pd{111} surface (Figure 3A) and single thiophene molecules (Figure 3B) in order
to characterize the electronic structure of the hydrogenation system prior to
reaction.� Although we observe effective
quenching of the Pd surface state by thiophene adsorption, we will continue
optimizing spectroscopic parameters in order to obtain reproducible and interpretable
single-molecule vibrational spectroscopy results.
�FIGURES Figures
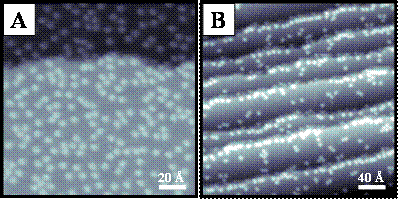
Figure
1. A) STM image (143 � � 143 �, Vs = 0.1 V, It = 150 pA) of molecular
thiophene adsorbed on a Pd{111} surface at 4 K.� Dosed after the Pd surface was allowed to
reach 4 K, thiophene appears randomly distributed on the surface.� The two regions seen in the image are atomic
terraces separated by a single Pd atomic step edge.� B) STM image (286 � � 286 �, Vs = -0.3 V, It = 100 pA) of molecular
thiophene aligned preferentially along the tops and bottoms of step edges on a
Pd{111} surface at 4 K.� Thiophene
was dosed at ca. 20 ‑ 40 K
and thus had some surface mobility on adsorption.
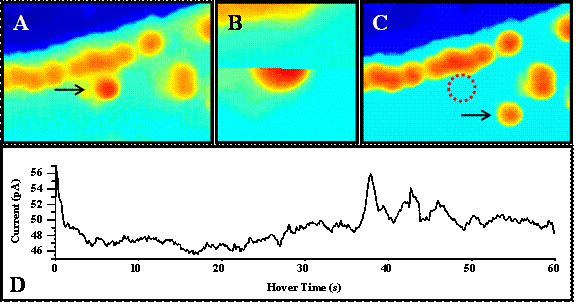
Figure
2. A) STM image (57 � � 38 �, Vs = -0.2 V, It = 100 pA) of molecular
thiophene adsorbed near a Pd{111} step edge at 4 K.� The arrow denotes a single molecule over
which action spectroscopy is performed.�
B) STM image (14 � � 14 �, Vs
= -0.2 V, It = 100 pA)
acquired before and after action spectroscopy.�
The thiophene moved out of the frame of imaging during spectroscopy,
resulting in what appears to be a partially imaged molecule.� C) STM image (57 � � 38 �, Vs =-0.2 V, It = 100 pA) after
action spectroscopy.� This STM image shows
the new location of the molecule from A.�
D) I vs. t spectrum (Vgap
= -0.35 V, Igap = 100 pA)
acquired over 60 seconds exhibits motion of the molecule under the STM tip
before finally moving out of the frame in B.
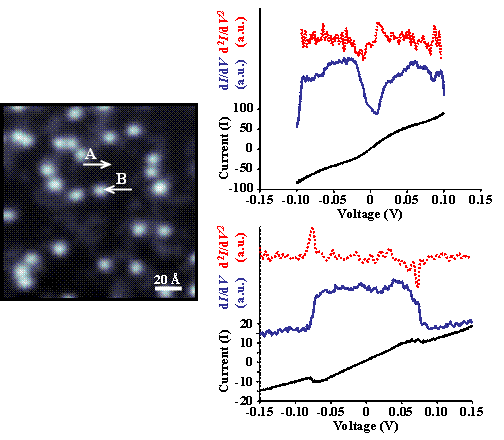
Figure
3. STM image (143 � � 143 �, Vs = -0.45 V, It = 40 pA) of molecular
thiophene adsorbed on Pd{111} at 4 K and accompanying spectra acquired over A)
Pd and B) thiophene (Vgap
= -0.01 V, Igap = 40
pA).� The broken red traces are the
result of mathematically differentiating the dI/dV spectra (blue).� The I/V response and subsequently the dI/dV
response of atomic Pd and molecular thiophene are markedly different.� A notable feature is the apparent quenching
of the surface state about the Fermi energy by the adsorbate.� Once parameters are optimized for IETS, single-molecule
vibrational spectra will be acquired simultaneously, enabling the assignment of
vibrational modes.
Back to top