Back to Table of Contents
46737-AC9
Multicomponent Droplet Growth in Supersonic Natural Gas Separators
Barbara E. Wyslouzil, Ohio State University
Natural gas supplies ~23% of U.S. energy needs. For off-shore
wells, treatment near the wellhead is critical to prevent clathrates
from forming and plugging the pipeline as gas flows to the mainland. The raw
gas is normally treated by adding chemicals or reducing its dew point, but
standard processing equipment is often large and requires manned platform
operation. An alternative approach is to use supersonic natural gas separators
that (1) cool the gas in a supersonic expansion to induce droplet formation and
growth, (2) separate the droplets from the gas, and, (3) recompress the dried
gas using a diffuser to minimize pressure losses. These separators are smaller
than traditional process equipment, have no moving parts, and require no
chemicals. Thus, they are suited for both off-shore and sub-sea applications.
As these devices are adopted,
critical questions remain regarding droplet growth in these complex vapor
mixtures. The goal of this work is to improve our fundamental understanding of
water - hydrocarbon droplet growth at cooling rates comparable of those found
in the novel separators. In particular, hydrocarbons can inhibit water
condensation because they wet the water surface while water cannot wet the
hydrocarbon surface. Since removing water is critical to preventing clathrate formation, understanding co-condensation in this
highly non-ideal system is vital to the success of supersonic separator
technology and efficient natural gas recovery.
Our experimental apparatuses include a series of supersonic nozzles with
cooling rates that match the supersonic separators. The characterization
methods include pressure trace measurements, infra-red spectroscopy
measurements of the gas phase, and in
situ small angle x-ray scattering measurements to directly follow the
growing droplets. To date we have made extensive measurements using the pure
components and limited experiments with the binary system. Figure 1 summarizes
the pressures and temperatures that correspond to the maximum nucleation rates
for a series of n-alkanes condensing in the nozzle.
Figure 2 illustrates some of the position resolved growth measurements for pure
nonane. In these experiments, the partial pressure of
the nonane at the inlet of the nozzle is fixed while
the total pressure of the Ar is increased by a factor
of two. The average particle size is larger in the low pressure case because
the heat released by the growing droplets quenches nucleation faster than when
there is more carrier gas available, fewer particles are formed, and these can
then grow to a larger size.
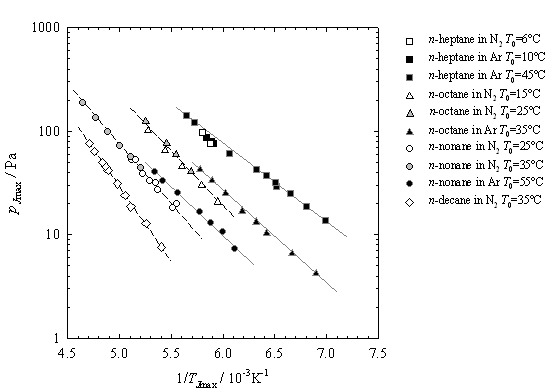
Figure 1. The conditions corresponding to the maximum nucleation rates for the
condensation of a series on n-alkanes follow
systematic trends: for a given carrier gas, N2 or Ar, the lines are parallel; at fixed temperature, the
pressure increases as the number of carbons in the alkane
decreases; for a fixed hydrocarbon partial pressure, expansions conducted in Ar condense at a lower temperature. The latter observation
is consistent with the fact that the cooling rate of the expansion is higher when Ar
is the carrier gas and, thus, we can probe the metastable
vapor region more deeply.
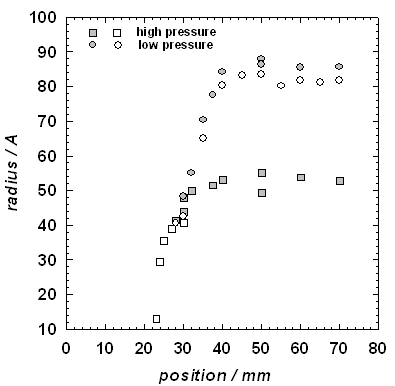
Figure 2. The average size of nonane
droplets increases rapidly as a function of position in the nozzle. We are able
to observe scattering from smaller droplets for the high pressure Ar experiments because the aerosol number density is
higher. The aerosol size distribution parameters are derived from fits of the
small angle x-ray scattering spectra to a Schultz distribution of polydisperse spheres.
Back to top