Back to Table of Contents
44110-G6
On the Photostability of Biomolecular Building Blocks and Their Clusters: The Role of pi sigma* States in Non-Radiative Decay
Susanne Ullrich, The University of Georgia
Exposure to ultraviolet (UV) radiation present in the sun's
spectrum or in artificial light sources is a major health risk due to adverse effects
such as sunburn, skin aging, and skin cancer. Among a number of other chromophores found in human skin, the deoxyribonucleic
acid (DNA) bases are strong absorbers of UV radiation. As the building blocks of
nucleic acids the photophysical and photochemical
properties of the DNA bases are implicated in the photostability of our
genetic coding material. To be effective, photoprotective deactivation
mechanisms must operate on ultrafast time scales in order to dominate over
competing photochemical processes that potentially lead to destruction of the
biomolecule. Using molecular beam techniques combined with
time-resolved spectroscopy, the excited state relaxation dynamics in isolated DNA
bases can be investigated in unprecedented detail free from interactions with
the surroundings or within a well-defined micro-environment (e.g. water clusters or base
pairing).
Femtosecond time-resolved
photoelectron spectroscopy (TRPES) provides unique capabilities for studying photoinduced processes in polyatomic molecules. Partial
ionization probabilities for ionization into cationic states of specific
electronic character can differ drastically with respect to the molecular
orbital nature of the neutral excited state. Hence a PES obtained via a two step excitation-ionization
scheme provides a distinct fingerprint of the neutral excited state. Changes in
the PES, observed as the delay between the pump and probe pulses is scanned,
can be associated with electronic configurational
changes during the relaxation process. This spectroscopic information only
obtainable through TRPES has proven crucial in discerning complex relaxation dynamics involving competing
deactivation pathways. Combined with time-of-flight mass
spectrometry using coincidence detection (PEPICO – photoelectron photoion coincidence) a TRPES spectrum of a certain
molecule/cluster size can be recorded even if a sample mixture is present in
the molecular beam.
Our experimental set up
consists of a fs laser
system that provides tunable UV pump and 200nm probe pulses and a magnetic
bottle-type PEPICO spectrometer. Two
time-delayed fs laser pulses interact with the
doubly-skimmed high intensity molecular beam (sample vapor seeded in He or Ar carrier gas) in the
ionization region of our PEPICO spectrometer. The photoelectron spectrometer uses a strong, highly divergent magnetic
field produced by an axially magnetized permanent ring magnet that meets a weak
guiding field to form a magnetic ‘bottle' allowing for a high collection
efficiency of photoelectrons. Ion detection is based on a modified Wiley-McLaren linear TOF mass spectrometer that
accommodates the ring magnet for the photoelectron spectrometer.
Time-resolved photoelectron
spectra of Adenine have been recorded at 246, 251, and 267nm excitation. The
two-dimensional data (see Fig. 1) was analyzed by simultaneously fitting the
decay dynamics and photoelectron spectra of the contributing relaxation
channels. For positive pump-probe delays a double exponential convoluted with a
Gaussian provides a good fit for the data. Energy integrated spectra including
these fits are shown in Fig. 1 (left attachments) and lifetimes are summarized
in Table 1. For all scans, the extracted time constant t1 is less
than 200fs and the lifetime t2 increases with longer pump
wavelengths. Photoelectron spectra associated with the longer time constant can
unambiguously be assigned to the S1 (nπ*) state; extraction
of the photoelectron spectra associated with the short time constant is
challenging as the fast pump-probe dynamics occur within the time-resolution of
our experiment. Spectral features overlap with those of the probe-pump signal
and hence their shape strongly depends on the fit parameters used for negative
delays. We have started a collaboration with the UGA
statistics department for further analysis of our data.
We
have also explored the applicability of our set up for studies of larger biomolecular building blocks. The time-of-flight mass
spectrum of Adenosine is shown in Fig. 1(d). Two different vaporization
techniques have been employed: simple heating inside our pinhole nozzle and a
pick-up source. Similar fragmentation pattern were observed with either
technique. We are currently investigating other possibilities for vaporization
of Adenosine, e.g. a spray jet nozzle.
Graduate
and undergraduate students have been actively engaged in setting up our new
laser laboratory and gained expertise in state-of-the-art techniques and
instrumentation of modern laser spectroscopy. S.U. and her research group have
greatly benefited from the ACS
PRF G Starter grant during these initial stages of establishing our independent
research program.
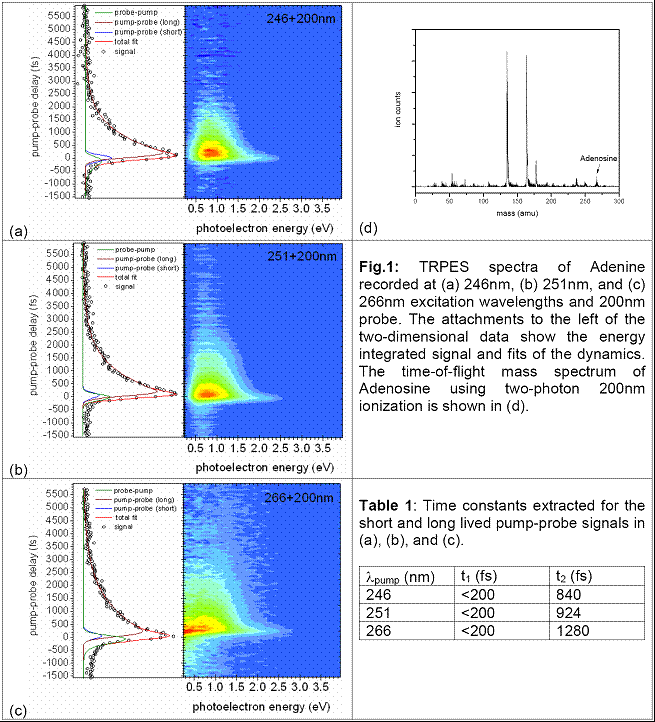
Back to top