Back to Table of Contents
44106-G6
Constrained Density Functional Theory as a Tool for Describing Electron Transfer
Troy Van Voorhis, Massachusetts Institute of Technology
In addition to
being among the most basic chemical processes known, electron transfer (ET)
reactions also play an active role in natural and artificial photosynthesis,
oxidative damage of DNA, respiration and single-molecule conductivity. In
particular, we are interested in how long
range ET is initiated and how the resulting electrochemical bias can be
used to do work. In this case, one
key factor is the reorganization energy
- the energy the molecule gains by distorting to accommodate ET. One would like to be able to make
quantitative predictions about the energy landscapes of these ET reactions
using first principles calculations, such as density functional theory
(DFT). Unfortunately, standard DFT
fails qualitatively for long range ET because of the lack of particle-hole
interactions in existing functionals. We have recently developed a method based
on constrained density functional theory (CDFT) that is well-suited to
describing long-range ET using diabatic states. Within this grant, we have proposed to build upon this basic
realization to build a consistent framework for the description of electron
transfer within DFT.
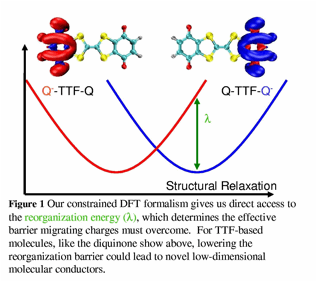
Year 1 Activity
In the first
stage of this grant, we have implemented CDFT gradients, which allow for rapid
molecular geometry optimization with a
fixed amount of ET. At a fundamental level, this allows us to explore the
diabatic potential energy surfaces involved in ET and also to address the
magnitude and nature of inner sphere reorganization within the reaction. We have already used this method to
predict the inner sphere reorganization in a few model donor acceptor systems,
and the resulting methodology has given us significant insight into charge
recombination in organic materials ADDIN EN.CITE <EndNote><Cite><Author>M.
Segal</Author><Year>2007</Year><RecNum>409</RecNum><MDL><REFERENCE_TYPE>0</REFERENCE_TYPE><REFNUM>409</REFNUM><AUTHORS><AUTHOR>M.
Segal, M. Singh, K. Rivoire, S. Difley, T. Van Voorhis and M.
Baldo</AUTHOR></AUTHORS><YEAR>2007</YEAR><TITLE>Extrafluorescent
Luminescence in Organic Light-Emitting
Devices</TITLE><SECONDARY_TITLE>Nature
Materials</SECONDARY_TITLE><VOLUME>5</VOLUME><PAGES>375-378</PAGES></MDL></Cite></EndNote>[1]. A simple example of an application funded by this proposal
is illustrated in Figure 1. Here,
the fundamental parameter (inner sphere reorganization) is predicted
quantitatively by our C-DFT calculations to be 13.3 kcal/mol – an
extremely high value, even for an organic molecule. In turn, this value indicates that the quinine-TTF-quinone compound shown is does not function as a very good
“molecular wire” as previously thought.
Our simulations allow us to account for solvent effects through the use
of established continuum solvation models and give us quantitative predictions
about the energy landscape of ET in chemically relevant molecules.
In the second
stage of this grant, we developed a means of computing the electronic coupling
between the diabatic surfaces described above. This coupling plays a significant role in determining the rate of electron transfer and whether
the reaction proceeds via an adiabatic or nonadiabatic
pathway. Our initial applications
of this technique showed that it gives accurate predictions of the coupling in
several prototypical situations.
By combining the gradients described above with this coupling
prescription one obtains a unified ab initio picture
of the kinetics of the electron transfer process.
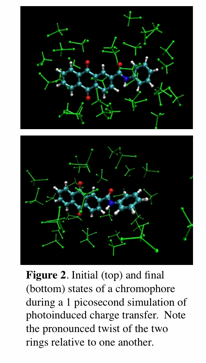
Year 2 Activity
Thanks to the
successful leveraging of this grant by an NSF CAREER award, we have at this
point accomplished all the major goals originally set out in our PRF
proposal. Moving forward, we have
extended this work by using the analytic gradients implemented within the PRF
grant to perform ab initio molecular dynamics simulations
of photoexcited ET. The new methodology is
implemented in the QChem program package and allows
for both fully quantum mechanical treatment of the potential energy surface as
well as QM/MM models. An illustration of the results that can be obtained with
this method are illustrated in Figure 2,
which shows the initial and final conformations of a molecule after photoinduced charge transfer. These simulations incorporate a quantum treatment of both
solvent and solute and display a characteristic twisted-internal charge
transfer (TICT) mechanims for the relaxation. This technique is very general and has
the capability of treating a wide variety of charge transfer reactions, from exciton quenching to redox
chemistry. At the same time, it is
still in its infancy and we are in the process of validating it against
experiment. We are grateful to the
funding from PRF that has allowed us to prime the pump on this exciting
project.
Back to top