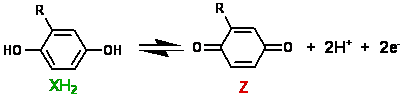
XH2/Z interconversion within NOM is usually assumed
to be reversible. Nevertheless, Michael-Type
nucleophilic addition by bisulfide ion (HS-), which renders the
reaction irreversible, has been documented:

Do weaker sulfur-, nitrogen-, oxygen-, and halogen-donor
atom nucleophiles form Michael-Type adducts?
Our hypothesis is that unactivated benzoquinones, i.e. those with
only alkyl substituents, cannot engage in reactions with weak nucleophiles,
while activated benzoquinones possessing electron-withdrawing
carboxylate substituents can. Carboxylate
substituents are abundant in lignin, an important NOM precursor
biomolecule. A number of physical
methods have demonstrated that NOM is rich in carboxylate groups. Carboxylate groups make dihydroxybenzenes
more resistant towards oxidation. In
order for this means of weak nucleophile incorporation to be viable, oxidants
strong enough to act upon carboxylate-substituted dihydroxybenzenes must exist
in marine environments.
Enrichments
of manganese(III,IV) (hydr)oxides have been found at
the oxic/anoxic interface within the water column of the Black Sea, within
marine sediments, and in a numerous other relevant environments. MnIII,IV
(hydr)oxides are strong enough in a thermodynamic sense and reactive enough in
a kinetic sense to oxidize carboxylate-substituted dihydroxybenzenes to activated
benzoquinones. We have investigated the
oxidation of more than sixteen phenols and dihydroxybenzenes by MnIVO2(s,
pyrolusite). Here are a few examples:
catechol (2.1x10-3 s-1) > hydroquinone (6.1 x10-4
s-1) > 2,5-dihydroxybenzoic acid (3.3x10-4
s-1) > 4,4'-biphenol (2.0x10-4 s-1) >
2,5-dihydroxyterephthalic acid (1.7x10-4 s-1) >
2,2'-biphenol (7.2x10-8 s-1). These values reflect initial rates of MnII
solubilization divided by the dihydroxybenzene substrate concentration,
measured in a 10 mM MES medium (pH 6.5).
Next, we
investigated adducts formed through attack by OH-/H2O, by
the nitrogen-donor nucleophile 4-methylimidazole, and by two sulfur-donor
nucleophiles, thiosulfate and sulfite.
Our efforts focused on HPLC with electrospray ionization (ES) mass
spectrometric (MS) detection, because of its' ability to identify specific
adducts. Capillary electrophoresis and
HPLC with UV detection yielded a similar number of peaks, but detection limits
were lower, and structure assignments were not possible.
As
expected, p-benzoquinone, classified as an unactivated electrophile,
formed an adduct with the representative sulfur-donor
nucleophile thiosulfate, but not with OH-/H2O or with 4-methylimidazole. p-Benzoquinone is
stable enough for a stock solution to be prepared from an authentic
standard. Thiosulfate or
4-methylimidazole was then added. With
ESI-MS, the ionization process is strong enough to generates the molecular ion
from -O3S-S-XH2 (the thiosulfate adduct), but
not from the p-benzoquinone substrate. HPLC with UV detection was used to monitor
concentrations of p-benzoquinone.
2,5-Dihydroxybenzoic acid oxidation by MnO2(s)
yields the activated electrophile carboxy-p-benzoquinone. In simple aqueous media (beginning pH ~ 5.6),
MnO2(s) oxidation followed by OH-/H2O
nucleophilic attack generated three addition products, and one product of
oxidative coupling, all shown to the left of the line, below.
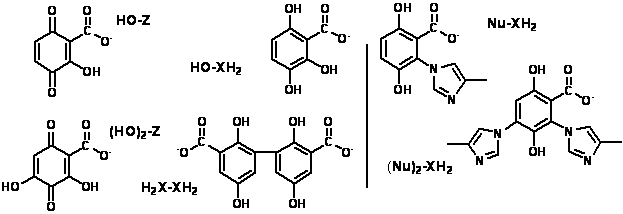
4-Methylimidazole is not subject to oxidation by MnO2(s),
so it can be added along with the 2,5-dihydroxybenzoic
acid substrate. 50, 100, 200, 300, and
400 mM
concentrations were employed. As the 4-methylimidazole
concentration was increased, peaks collected in total ion mode for the left
four species to decreased, and new peaks corresponding to the 4-methylimidazole
adducts (to the right of the line) increased.
MS fragmentation patterns are consistent with the structural
assignments. Sulfite ion and iodide are
subject to oxidation by MnO2(s).
It was therefore necessary to first generate carboxy-p-benzoquinone
using MnO2(s), then remove unreacted MnO2(s)
prior to addition of nucleophile stock solution using microfiltration. Sulfite ion yielded an
adduct discernible by HPLC-ESI-MS; iodide did not.
When 2,5-dihydroxyterephthalic acid is oxidized by MnO2(s),
a highly activated electrophile is generated, dicarboxy-p-benzoquinone. With have conducted experiments employing 50,
100, 200, 300, and 400 mM 4-methylimidazole, and discerned the following
products using HPLC-ESI-MS:
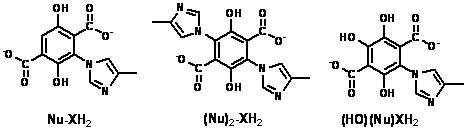
The rightmost product, a mixed hydroxide
ion/4-methylimidazole adduct, is particularly noteworthy. It's peak area
reached a maximum value when 200 mM 4-methylimidazole was employed.
We have
several important objectives for the second and final year of this
project. We will expand our structural
determinations to include oxygen-donor and halogen-donor nucleophiles
representative of marine sedimentary environments. We are also interested in whether other
transient species generated by oxidation, e.g. quinone methides, provide
additional opportunities for adduct formation.
We will employ reactions performed at higher concentrations (~ 10 mM) to
generate enough quantities of each product for isolation and purification to be
conducted. Once we have authentic
standard prepared, we can quantify production rates and compare rate constants
for OH-/H2O adduct formation with rate constants obtained
with nucleophile amendments. We are
currently testing "Good's Buffers" such as MES and MOPS to see if
they are sufficiently nucleophilic to generate Michael Addition products. Once we have identified an inert buffer
system, we can carefully work out rates of competing adduct formation reactions
within a range of pH appropriate for marine sedimentary environments.
Back to top