Back to Table of Contents
46425-AC3
Understanding Iron Catalyzed Olefin Polymerization
Paul J. Chirik, Cornell University
As outlined in our original proposal, the goal of our
PRF-funded program is to elucidate the nature of the active species and provide
insight into the mechanism of iron-catalyzed olefin polymerization. Ideally,
the information gleaned from these studies will be used to design more active
and selective catalysts for polyolefin production. As the price of crude oil
continues to skyrocket, more efficient use of petroleum feedstocks such as
ethylene and propylene becomes essential for the stability of the domestic
economy.
Unlike more traditional titanium and zirconium Ziegler-Natta
polymerization catalysts where the mode of propagation and nature of the active
species is well-established, the potential for multiple oxidation states in
iron chemistry has spawned controversy over the
the identity of the true catalyst for over a decade. During
our first year of PRF support, our laboratory has focused on preparing
functional models of the propagating iron species as well as initiated
fundamental studies into the stability of the iron-carbon bond.
Our laboratory had previously reported the synthesis of
bis(imino)pyridine iron dialkyl complexes and their activation with borane and
borate reagents to yield the first examples of single component iron catalysts
for the polymerization of ethylene. During the past year, we have studied the
polymerization reaction in more detail by 1H NMR spectroscopy and
discovered that catalyst initiation was much slower than propagation (Figure
1). As a result, only the starting iron alkyl cation, ethylene and polymer are
observed during turnover, providing no information about the nature of the
active species, rate of propagation, termination, etc.
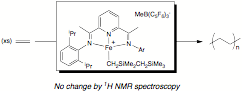
Figure 1. Synthesis and ethylene polymerization activity of
bis(imino)pyridine iron alkyl cations.
Based on these findings, we sought to replace the Fe-CH2SiMe3
or Fe-CH2SiMe2CH2SiMe3 groups with
more polymerization relevant alkyls containing b-hydrogens, e.g. Fe-CH2CHR2. While
attractive from a mechanistic standpoint, this is an extremely challenging
synthetic proposition as many common alkyating agents (RLi, etc) serve to
reduce rather than alkylate the iron center and the bis(imino)pyridine chelate. Because of these challenges we sought to find new
methods for the preparation of neutral bis(imino)pyridine iron alkyl complexes
that could serve as synthons, via oxidation, to the desired cationic,
catalytically competent alkyl complexes. This strategy has already proven
successful for b-hydrogen stabilized alkyls such as neopentyl.
We discovered that oxidative
addition of various alkyl bromides to the bis(imino)pyridine iron
bis(dinitrogen) complex, 1-(N2)2, served as a convenient method for the synthesis of
various alkyl compounds containing b-hydrogens (Figure 2). In this manner, the first
examples of long sought after bis(imino)pyridine iron ethyl, n-butyl, n-hexyl,
isobutyl and methylcyclopentyl compounds were prepared and characterized.
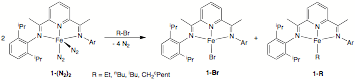
Figure 2. Synthesis of bis(imino)pyridine iron alkyl complexes
containing b-hydrogens.
The kinetic stability of each 1-R compound was assayed in benzene-d6
solution and found to produce a mixture of the corresponding alkane and alkene.
The kinetic stability of the iron alkyl complexes was inversely correlated with
the number of b-hydrogens present. For
example, the iron ethyl complex, 1-Et,
underwent clean loss of ethane over the course of hours, while the
corresponding 1-iBu compound
had a half-life of over 12 hours under identical conditions (Figure 3). The
mechanism of the decomposition was studied with a series of deuterium labeling
experiments and supports a pathway involving initial b-hydrogen elimination followed by
cyclometalation of an isopropyl methyl group, demonstrating an overall transfer
hydrogenation pathway. The relevance of this unexpected decomposition pathway
to cationic compounds active for polymerization is currently under
investigation in our laboratory.
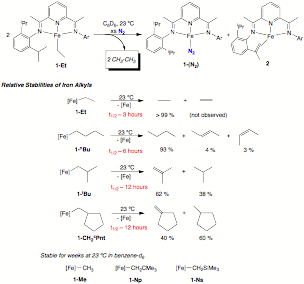
Figure 3. Decomposition pathway for bis(imino)pyridine iron
alkyl complexes containing b-hydrogens and
relative half-lives as a function of alkyl group.
A second major area of focus during the past funding period
has been the elucidation of the electronic structure of bis(imino)pyridine iron
dialkyl, neutral monoalkyl and cationic alkyl complexes. Such data may
ultimately prove essential for assigning the oxidation state and degree of
chelate participation for industrially relevant catalysts activated with
methylaluminoxane (MAO). For the bis(imino)pyrdine iron dialkyl, 1-Ns2
(Ns = CH2SiMe3),
metrical parameters from X-ray crystallography suggest one electron reduction
of the chelate, implying a ferric center antiferromagnetically coupled to a
ligand radical anion. To further explore this possibility, SQUID magnetic and
zero field Mssbauer data were collected (Figure 3). Fitting the magnetic data
yielded a zero feilf splitting parameter (D) of 6.8 cm-1
and a g = 2.008. These values, in combination with the Mssbauer isomer shift
of 0.27 mm/sec, support high spin iron(III). Similar studies are ongoing with
the corresponding alkyl cations. X-ray and Mssbauer data (d = 0.64 mm/sec) have been collected and may
resolve some of the conflict in the literature over the role of ferrous versus
ferric centers during chain propagation.
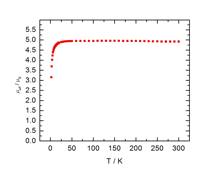
Figure 3. SQUID magnetic data and zero field Mssbauer spectrum
of 1-Ns2.
Back to top