Back to Table of Contents
45727-G9
Liquid Transport Mechanisms and Surface Interactions in Nanoporous Materials
Geoffrey D. Bothun, University of Rhode Island
The goal of
this project is to elucidate liquid-surface
interactions within nanoporous materials and examine how these interactions
influence transport behavior.
Nanoporous ceramic membranes are excellent candidates for solvent-based
separations as they are solvent inert, stable to high temperatures, and can
accommodate high transmembrane pressures and complex multicomponent solvent
feeds. One factor that limits the
applicability of nanoporous ceramic membranes for such separations is
unpredictable solvent transport behavior due to strong interaction between the
solvent and the walls of the nanopores.
This is not the case for macroporous membranes where the pore diameters
are much greater than the length scales for these interactions. Solvent-surface interactions depend on
the chemical and physical properties of both the solvent and membrane, and are
difficult to predict a priori. There are currently no ubiquitous
models that can account for the effect of non-specific liquid-phase solvent
interactions on nanoscale fluid mechanics.
An additional component of this project is to train
undergraduate students from underrepresented groups and enhance professional
development skills. This project
has directly supported two undergraduate researchers, and the research
activities of a female high school intern (summer 2007). One of the undergraduates was a female
student, and the research she has conducted has led to a manuscript in press
for the Journal of Membrane Science.
She will be a co-author on this publication, which is an excellent
accomplishment for an undergraduate research assistant. She has also given two research presentations.
Solvent-surface
interactions and solvent transport in nanoporous ceramic membranes
Extensive progress has been made to characterize solvent-dependent
transport and the role of surface interactions in commercial nanoporous ceramic
membranes using permeability and thermoporometry measurements. The membranes
chosen were titania (TiO2) with tortuous interconnected pores (1, 5,
and 50 kDa, corresponding to pore diameters of ca. 8.2, 18.3, and 33.2 nm,
respectively) and alumina (Al2O3) with non-tortuous 20 nm
cylindrical pores. A novel pre-water/solvent/post-water permeability cycle was
employed to account for structural differences between membranes and to gauge
the effect of residual solvent on water permeability at different temperatures. Solvent-dependent interactions were
represented by a lumped parameter,
, that describes solvent permeability relative water. These values were determined
experimentally using the following expression:
(4)
where J is the
solvent flux, h is solvent viscosity, DP is the transmembrane pressure,
and
and
are the solvent and water permeability coefficients,
respectively.
The effect of pore size on solvent transport was shown by
comparing water, 1-butanol, and cyclohexane permeability in 1, 5, and 50 kDa
titania Sterlitechª membranes (Figure 1).
For both 1-butanol and cyclohexane, there is a decrease in the
normalized permeability, a (solvent/pre-water), with decreasing
pore size at each temperature.
However, the normalized permeability coefficients for 1-butanol were much
lower than that of cyclohexane in the 1 and 5 kDa membranes, suggesting that
surface interactions associated with 1-butanol have a greater impact on
restricting transport relative to water.
Increasing temperature did not have a significant effect on 1-butanol
permeability relative to water in the 1 and 5 kDa membranes, but it did cause a
reduction in cyclohexane permeability.
Similar effects were observed in the 20 nm alumina membranes.
Auger spectroscopy revealed that 1-butanol chemisorbed on
the membrane surface, which led to a ca. 0.5 nm reduction in the effective pore
size and created a hydrophobic surface.
Post-water runs revealed that this modified hydrophobic surface restricted
water flow. Cyclohexane
physisorbed on the membrane surfaces and, because of its spherical structure,
organized along the surface to increase the disjoining pressure, which reduced
the effective pressure driving force (DP).
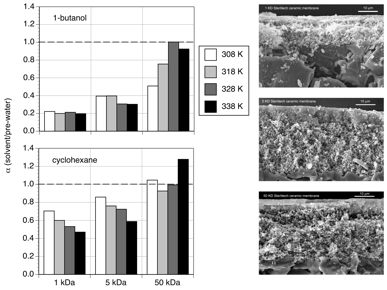
Figure 1. Normalized 1-butanol and cyclohexane permeability as a
function of temperature through 1, 5, and 50 kDa titania membranes. Solvent permeability was normalized
against initial water permeability measurements, a (solvent/pre-water) =
. SEM images
reveal the separation, intermediate, and support layers of the 1 (top), 5
(middle), and 50 kDa (bottom) membranes.
Through this study we have shown that solvent
viscosity alone cannot describe permeability in nanoporous ceramic membranes. The potential for chemical and physical sorption,
and the nature of the solvent layer at the pore wall greatly impact transport. Additional work is being conducted
using thermoporometry to elucidate solvent-surface interactions in native
membranes and hydrophobic membranes surface modified with alkyl and fluoroalkyl
silanes.
Back to top