Back to Table of Contents
44891-B3
Preparation, Characterization, Film Fabrication, and Photoluminescence of Metal-Organic Networks of Copper(I) Cyanide
Robert D. Pike, College of William and Mary
The major finding
from last year that copper(I) cyanide forms diamine
and diimine networks has been further confirmed and expanded. The networks of CuCN with N-substituted piperazines (e.g., Me2Pip = N,N′-dimethylpiperazine, MePip = N-methylpiperazine, EtPip = N-ethylpiperazine, Ph2CHPip = N-diphenylmethylpiperazine) and hexamethylenetetramine (HMTA),
among others, have been studied. These diamine and tetramine
ligands (B) produce stable, highly luminescent (CuCN)n(B)
networks self-assembled in single-step, high-yield, aqueous reflux reactions of
CuCN and B in the presence of KCN.
A common
structural theme is the formation of (CuCN)2B
hexagonal honeycomb 63 networks, which consist of tiled Cu6(CN)4B2
units as a result of bridging B and mu2-cyano groups. This motif is
very common with piperazine networks of CuCN, such as
(CuCN)2(Me2Pip),
Figure 1A. The 63 network pattern is also found threaded with additional
CuCN chains in relatively copper-rich phases, such as
(CuCN)7(B)2
= 2[(CuCN)2B]�3CuCN (B = MePip, EtPip). In Figure 1B the 63
network is viewed edge-on and is illustrated with its Cu atoms colored orange;
the threaded CuCN chains are shown with Cu atoms
colored green. (In all X-ray figures organic ligands are shown as wireframes
and all cyano C/N atoms are colored grey to reflect site disorder.) CuCN-threaded networks are also noted for (CuCN)4(B) = [(CuCN)2(MePip)]�2CuCN
(B = Me2Pip, Et2Pip). Non 63 network motifs
are seen for (CuCN)n(HMTA) (n = 1.5, 2.5, 5) and (CuCN)2(Ph2CHPip), as illustrated in
Figures 1C and 1D. The HMTA compounds show extensive mu3-cyano
bridging and the Ph2CHPip compound reveals a CuCN
chain uniquely decorated with -CN-B units.
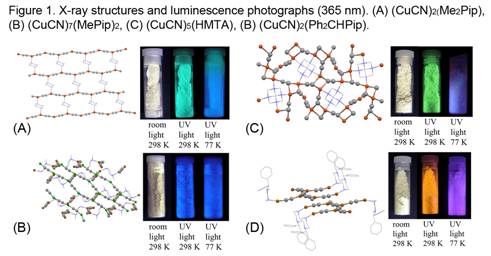
The new copper(I) cyanide networks show interesting spectroscopic
behavior. CuCN itself is colorless, absorbing in the
UV (see Figure 2A), but luminesces intensely at the border of the UV and
visible. The new diamine and tetramine networks are more-or-less white but in
many cases are highly luminescent. This phenomenon is currently being investigated
collaboratively by the P.I. with Prof. Howard Patterson (University of Maine,
variable temperature spectroscopy) and Prof. Craig Bayse
(Old Dominion University, computational analysis). The major findings are:
1)
Density Field Theory (DFT) calculations suggest that
excitation in CuCN involves p MOs combining metal (3d to 4p) and cyano (pi to pi*)
contributions. Excitation is thus an admixture of metal-centered (MC) and
metal-to-ligand charge transfer (MLCT) transitions. Hence, the oft-made
distinction between MC and MLCT is probably not meaningful for CuCN networks.
2)
CuCN-amine emission is red
shifted and broadened with respect to that of CuCN
(Figure 2). Additionally, fine structure is sometimes apparent in CuCN-amine excitation and emission (see Figure 2C). In
threaded networks (e.g., see Figure 1B) the emission fine structure appears to result
from a combination of CuCN (wavelength = 412 nm) and CuCN-amine (wavelength = 450 nm) components.
3)
An additional low-energy, thermochromic emission band in
the yellow-orange region is sometimes present (see Figures 1A, C, D and 2D).
The origin of this band is not yet understood.
4)
Time-Dependent DFT results suggest that the triplet
state is lowered in energy via bending of the CuCN at
copper. Since this effect opens a potential coordination site, it may ease
reaction with incoming nucleophiles, facilitating exciplex formation.
Conversely, bent CuCN-amine complexes should be
expected show lower energy emission than does CuCN,
as is experimentally observed.
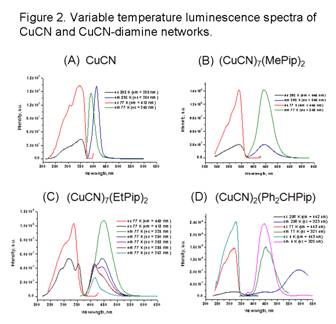
Research has
been initiated on the luminescence of monoamine (L) adducts of CuCN. It has been found that addition of small amounts of
liquid or vapor-phase amine to solid CuCN causes
luminescence emission to shift from the near UV value of 392 nm characteristic
of CuCN to wavelengths well into the visible. Figure
3 reveals the varied effects of a variety of liquid amines on the luminescence
emission of CuCN. As shown in Figure 3, slight
chemical changes can produce the significantly different emission colors, e.g., green for CuCN/piperidine
(Figure 3G), yellow for CuCN/N-methylpiperidine (3H)
and pink for CuCN/N-ethylpiperidine (3I). The results
of thermogravimetry and X-ray powder diffraction have
indicated the following facts:
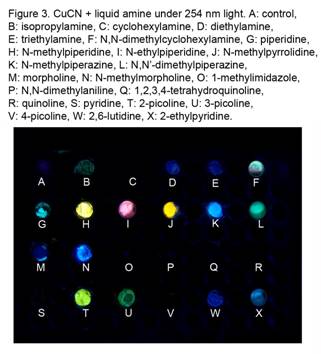
1)
Exposure of CuCN to amine
liquid or vapor produces a surface coating of a new luminescent chemical phase
that corresponds to only a few mass percent, with the bulk remaining CuCN.
2)
In each case, the new luminescent phase matches the
authentic CuCN-L structure (when the latter is
known).
3)
Eight CuCN-L phases are
known, two of which have been solved in our lab, see Figure 4. Each consists of
infinite CuCN chains decorated with 1-2
amines/copper, resulting in stoichiometries of (CuCN)(L)q (q = 1.0, 1.33, 1.5, 2.0).
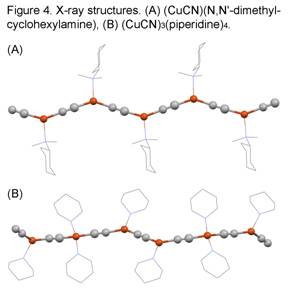
����������� These
findings are of interest with regard to potential chemical sensors for volatile
organics in the environment. Based on the above results, a putative �sniffing�
device based on CuCN would be able to distinguish
closely related N-alkylpiperidines. It would also be
able to distinguish 2-, 3-, and 4-methylpyridine.
Back to top