Back to Table of Contents
43706-AC9
Connecting Catalytic Chemistry to External Particle Conditions via Computational Fluid Dynamics
Anthony G. Dixon, Worcester Polytechnic Institute
The objective of
this project is the more realistic
treatment of catalyst particle internal transport and reaction processes by
linking them to conditions in the fluid flowing past and surrounding the
particle. The work is divided into two main tasks: 1) development and testing
of computational fluid dynamics (CFD) approaches to coupling intraparticle
chemistry and species transport to the external flow field; 2) evaluation of
the behavior of catalyst particles near the wall of a steam reformer reactor
tube, and the development of improved particle models.
Our original proposal for porous particles
in the CFD simulations was to treat them as solid regions and write
user-defined code to include species and reactions in the solid. This was very
time-consuming, and we devised a different approach, defining the particles as
porous regions for which species mass fractions were available. In the interim,
however, Fluent released version 6.3, which provided user-defined scalars (uds) in solid regions. We were then able to take advantage
of these new developments in the CFD code to re-visit our original approach of
modeling particles with solid regions. Our motivation for doing this was to
resolve a small problem that arose when we looked in detail at the fluxes into the
porous particles. We found that with the porous region approach, there was a
residual convective flux into the particle, which was an artifact of the
discretisation method. Although small, this flux carried enough enthalpy to
increase the particle temperature significantly, over what was expected
compared to benchmark calculations.
We developed a uds solid particle approach, which
uses species mass balances and energy inside the solid particles, and the standard
CFD equations for uds in the gas phase. The gas-phase
uds are then coupled to the mass fractions in the
gas, which ensures that the change in moles in the gas phase due to reaction is
properly captured. We have carried out a series of validation studies, in which
the new method was used on simplified situations: a) gas phase reactions only,
where we compared to the built-in reaction models of Fluent; b) solid sphere
isothermal 1st-order reaction for which an analytical solution was
available; c) constant known heat and mass sources inside the particle, to
check that the correct particle-gas transfer took place. We have repeated our
earlier work on simulation of wall segments of spheres and full cylinders, using
the new validated method. Comparison to the porous region approach results that
were reported previously, showed that all qualitative results and trends were
unaffected, only the temperature profiles decreased in magnitude. We extended
the analysis of these simulations to look more closely at regions of reactant
depletion between particles.
Previously, we developed meshes, and conducted
flow simulations, around single particles inclined at different angles to the
oncoming flow, for complex particle shapes with multiple holes. In the present
reporting period, we extended these simulations to include heat transfer,
diffusion of species and reaction in the particle, using the user-defined
scalar solid particle approach described above. This work has allowed us to
evaluate the surface accessibility to flow, and thus
reactants, of internal voids, and how this depends on angle of attack. We found
that both methane (reactant) and heat uptakes were at a maximum at an angle of
approximately 30° to the flow. Plots of heat and species fluxes for the
individual surfaces of the particles showed complex interactions with flow. As the
upstream flat and inner hole surfaces turned from
being directly exposed to the flow, their effectiveness decreased. The outer
curved surface changed from boundary layer flow over the whole area, to flow
directly impacting on the upstream part of the surface, with a strong
recirculation flow on the downstream part. The overall effectiveness of the
surface increased, and the asymmetry of temperature and species in the particle
also increased. It will be important to see to what extent these single
particle results carry over to a bed of particles, as such strong variations
within a particle would have implications for uneven deactivation through
carbon deposition.
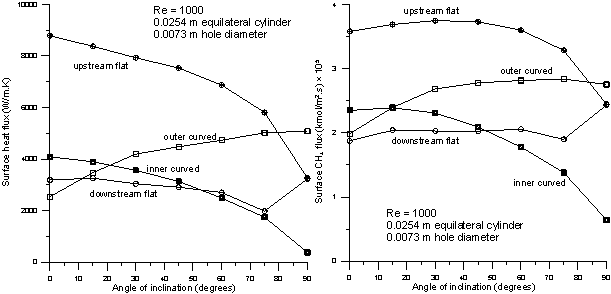
The funding provided by this ACS/PRF
grant partially supported Dr. Ertan Taskin in a postdoctoral stay following his Ph.D. defense
last year. Mr. Alexandre Troupel
arrived last September, was employed as a TA through the academic year by the
department, joined the research project in November, and was released by the
department to become an RA in May. The PI received a SUMR supplement, which was
used to support Ms. Winnie Nabea over the summer. Her
work has provided us with a heat transfer experimental facility for validation,
and she evaluated AUTOCAD, an alternative method of generating solid packing
models for meshing. The ACS/PRF funding continues to allow the PI to develop
the more chemistry-based aspects of the CFD research effort, which promises
well for future applications.
Back to top