Back to Table of Contents
46636-AC2
Quantum Mechanical Calculation of Hydrogen Isotope Exchange Thermodynamics and Kinetics on Petroleum Model Compounds
James D. Kubicki, Pennsylvania State University and Katherine H. Freeman, Pennsylvania State University
The first year of research into
the thermodynamics and kinetics of H-D isotopic exchange on kerogen-related
molecules was conducted by performing tests on the accuracy of the
computational methodology employed, modeling a range of H sites within molecules,
and calculating fractionation factors on alkanes of various molecular
weights.� This research will be used to
help interpret observed H-isotope fractionation factors in ancient organic
matter from sedimentary rocks.�
Theoretical values are needed because molecular-specific isotopic
analyses have recently been made possible, but experimental values are
difficult to obtain especially for specific sites within compounds.� Observed fractionation trends will require
knowledge of both the equilbrium fractionation factors and exchange rates as a
function of temperature.
The compounds 2-methylbutane,
2,6-methyloctane, pristane and phytane were chosen because they all contain at
least four distinct H sites (both a-
and b-primary, secondary and tertiary �
Figure 1).� Thus, one can predict the
relative fractionation factors and exchange rates for different site to
determine whether molecular structure will affect the total H-D isotopic
exchange with water.� Previous work
(Pedentchouk et al., 2006) has shown that branched alkanes undergo
significantly more H-isotope exchange; thus we hypothesized that the
fractionation factors may be greater and/or the exchange rates faster for
tertiary sites than for primary sites.
Our first set of results confirms
this hypothesis.� For example, Table 1
shows the equilibrium fractionation factors, a-values,
for our suite of compounds as a function of site within each compound.� Equilibrium exchange is consistently
predicted to be significantly greater at tertiary sites compared to primary
sites (i.e., smaller a-values) by 50 to
70 per mil.
Table 1 � Calculated fractionation factors energies for
a suite of test compounds as calculated with quantum mechamical methods.� Free energy (DG)
values are the calculated against H2O and the DG's are the differences between reactants
and products (HDO + RH � H2O + RD where R is the organic compound).
�a-values
were determined from the DG.� The units for DG are in kJ/mol.� The temperature was 298K.
____________________________________________________________________________
Molecule/Method������������� a-primary������������������� b-primary��������� Secondary��������� Tertiary
������������������������������������������� DG���� a������ DG�������� a������ DG�������� a������ DG�������� a__�����
2-methylbutane
CBS-Q������������������������������� +0.24 0.907�� +0.22����� 0.915�� +0.03����� 0.988�� -0.10������ 1.043
MP2/6-31G(d,p)���������������� +0.93 0.678�� +0.94����� 0.684�� +1.10����� 0.643�� +1.17����� 0.624
MP2/6-311++G(d,p)���������� +0.95 0.683�� +0.99����� 0.672�� +1.09����� 0.645�� +1.17����� 0.625
B3LYP/6-31G(d,p)������������ +0.98 0.673�� +0.96����� 0.680�� +1.15����� 0.629�� +1.22����� 0.611
B3LYP/6-311++G(d,p)������ +1.10 0.642�� +1.07����� 0.650�� +1.24����� 0.606�� +1.31����� 0.590
2,6-methyloctane
MP2/6-31G(d,p)���������������� +1.03 0.660�� +0.98����� 0.673�� +1.13����� 0.633�� +1.24����� 0.606
B3LYP/6-31G(d,p)������������ +1.07 0.649�� +1.00����� 0.667�� +1.16����� 0.627�� +1.35����� 0.580
B3LYP/6-311++G(d,p)������ +1.12 0.635�� +1.06����� 0.652�� +1.19����� 0.619�� +1.38����� 0.574
Pristane
B3LYP/6-31G(d,p)������������ +1.01 0.665�� +1.02����� 0.663�� +1.16����� 0.626�� +1.26����� 0.601
B3LYP/6-311++G(d,p)������ +1.03 0.661�� +1.07����� 0.648�� +1.22����� 0.611�� +1.30����� 0.592
Phytane
B3LYP/6-31G(d,p)������������ +1.08 0.648�� +1.07����� 0.649�� +1.19����� 0.619�� +1.30����� 0.591
B3LYP/6-311++G(d,p)������ +0.96 0.680�� +1.02����� 0.663�� +1.11����� 0.639�� +1.22����� 0.611
_____________________________________________________________________________
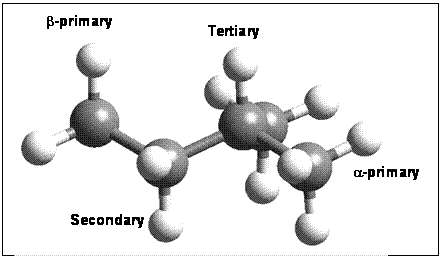
Figure 1 �
2-methylbutane molecule showing various types of H sites undergoing isotopic
subsitution.� Frequency anlalysis with H
or D in each position gives the DG
values presented in Table 1 while energy minimizations with one H abstracted
from each site provides the energies in Table 2.
By calculating the Gibbs free
energies of H abstraction energies at each site as well, we have estimated the
activation energy barrier to H-isotope exchange.� These calculations were performed with and
with H2O and the results listed in Table 2. In general, the model H
radical abstraction energies are 20 to 30 kJ/mol lower
for tertiary sites compared to primary sites.�
Thus, at temperatures found in many organic-rich sediments of interest
(50 to 100�C), the rates could be different by as much as six orders of
magnitude.� Obviously, this complicates
interpretations of molecular-specific H-isotope analyses because equilibrium
fractionation may be obtained at some sites and not at others.� Accurate temperature estimates for the
sediments will be required in order to deconvolute this signal.� The reactions modeled with H2O
present decreased the H+ abstraction energies by
approximately 100 kJ/mol which has a dramatic effect on predicted rates.� Lewan (1997) has shown that water dissolved
in bitumen is capable of exchanging H-isotopes with organic compounds, so the
presence and isotopic signal of any water associated with sedimentary organic
matter must be considered when interpreting the H-isotope signal.
Table
2 � Activation barrier energies for the H abstraction from 2-methylbutane
and water reaction.� The energies
are in kJ/mol.� The reaction for the
activation barrier energy was 2MeBut + H2O � 2MeBut- + H3O+.�
_____________________________________________________________________
Molecule/Method����������������������� a-primary��� b-primary���� Secondary Tertiary____
MP2/6-31G(d,p)������������������������������ +349������������ +276������������� +262���������� +272
MP2/6-311++G(d,p)����������������������� +326������������ +368������������� +305���������� +298
B3LYP/6-31G(d,p)�������������������������� +317������������ +283������������� +228���������� +288
B3LYP/6-311++G(d,p)������������������� +315������������ +283������������� +227���������� +286
_____________________________________________________________________
References
Pedentchouk N., Freeman K. H.,� Harris N. B. (2006)
Different response of dD values of n-alkanes, isoprenoids andkerogen during
thermal maturation Geochimica et Cosmochimica Acta, 70, 2063-2072
Lewan M.D. (1997)
Experiments on the role of water in petroleum formation. Geochimica et Cosmochimica Acta, 61, 3691-3723.
Back to top