Back to Table of Contents
44879-AC5
Direct and In Situ Investigation of Molecular Relaxation and Transport Properties of Nanocomposite Polymer Materials
Rene M. Overney, University of Washington
We are investigating the relationship between polymer mobility and local gas flux in heterogeneous polymer membranes. Our efforts focus on poly(trimethyl silyl propyne) (PTMSP)-silica nanocomposites that exhibit high permeability and high reverse selectivity, both of which are improved by increased silica concentration.1,2 With non-scanning resistively heated atomic force microscopy (HT-AFM), we probed for thermomechanical relaxations (transitions) in PTMSP. Thereby, material transitions at 330 °C could be attributed to molecular degradation. With scanning HT-AFM at a fixed probe temperature, critical nanoparticle-polymer delaminating shear forces could be determined as function of temperature, load and scan velocity. With increasing temperature, the probe particle impact force in PTMSP showed an abrupt change at T'= ~300 °C, indicating an interfacial transition below bulk degradation (figure 1). Above the interfacial transition temperature, impact forces increased significantly and ultimately resulted in particle debonding at Tdeb= 326 °C, as shown in figure 2. The interfacial transition suggests enhanced polymer mobility at the interface, which is in accordance with the improved permeability of the nanocomposite. Debonding was found to occur more readily in smaller diameter particles (<60nm) than in large diameter particles (>100 nm). A debonding energy per unit area of 2.6 J/m2 is deduced from the particle height, subsequent cavity depth and the impact force of debonding. It was found to exceed, by an order of magnitude, the work of adhesion between a planar and ultrasmoogh system of silica and polymethyl methacrylate (PMMA). These high debonding forces are possible caused by the surface roughness and curvature effects.
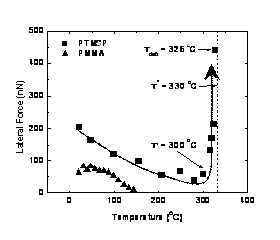
Figure 1: Lateral force vs probe temperature on silica particles embedded in PTMSP (°) and PMMA ().
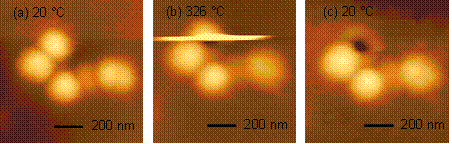
Figure 2: Detailed debonding scans on PTMSP at (a) room temperature, (b) 326 °C, and (c) room temperature after scanning.3
Of particular interest is the impact of interfacial thermomechanical properties on gas flux properties of the silica-PTMPS nanocomposites. In particular, knowledge of the size of the interfacial domain, and the extent of permeability enhancement, would help in optimal design of the nanocomposite membranes. Much like an air hockey table, gas flux through a membrane reduces surface friction, therefore microscopic measurements of friction are sensitive to local changes in the gas flux.4 Based on friction force microscopy, we have developed a sub-micron local flux measurement technique, also referred to as flux lateral force microscopy (flux-LFM), that provides the friction force as a function of the inlet gauge pressure, Fig. 3(a). Prior to applying the technique to PTMSP, which exhibits solution-diffusion behavior, we have used the purely diffusive flux of permanent gases through a porous anodisc as a model membrane. Figure 3a shows friction vs. pressure plots for H2, N2 and CO2 through a 200nm pore size anodisc. Steeper slopes correspond to faster gas flux. Fig. 3(b) shows a linear correlation between the friction-pressure gradient and the bulk permeability. Friction-pressure gradients on successively smaller scans from 5 μm to 50 nm show a major inflection below 200nm, corresponding to the porosity of the membrane. By applying this technique to PTMSP nanocomposites, our goal is to determine heterogeneous fluxes, i.e., the flux profile from the polymer matrix to the particle interface.
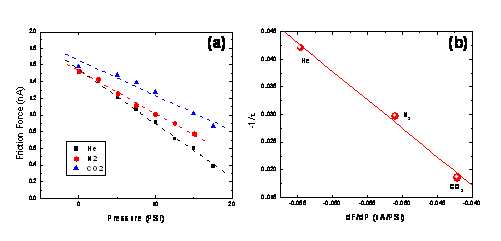
Figure 3: (a) Flux-LFM friction vs pressure for He, N2 and CO2 in a 200 nm Anodisc.
(b) Correlation between bulk permeability and friction-pressure gradient.
References
ADDIN EN.REFLIST 1. Nagai, K. et al., Progress in Polymer Science 2001, 26, (5), 721-798.
2. Merkel, T. C. et al., Macromolecules 2003, 36, (18), 6844-6855.
3. Gray, T. et al., Nanotechnology 2007, 18.
4. Wei, J. et al., J. Membrane Sci. 2006, 279, 608-614.
Back to top