Back to Table of Contents
46305-AC7
Surface Grafted Poly(ionic liquid)s
Scott M. Husson, Clemson University
Our goal
is to develop methodologies to create poly(ionic liquid) (pIL) nanolayers.
Program objectives are to prepare pIL nanolayers by surface-initiated atom transfer radical polymerization (ATRP),
characterize the nanolayer structural properties and growth kinetics, and
conduct fundamental thermodynamic property measurements to evaluate pIL
nanolayer performance for selective sorption of CO2. Results from
our research will enable the design of membranes with ultrathin pIL separation
layers to recover CO2 emissions generated by combustion of fossil
fuels, thusly lessening the effect of global climate change.
Proposed
activities for Year 1 were these:
1. Synthesize IL monomers and develop the
methodology to prepare pIL nanolayers on silicon substrates and silica beads.
2. Characterize the chemical and structural
properties of the pIL nanolayers using FTIR, ellipsometry, AFM, DSC, and GPC.
[proposed for Years 1-2]
Additional
activities that we did in Year 1 were these:
3. Evaluate how the chemistry of pIL
nanolayers impact their performance for selective sorption of CO2
over N2. [proposed for Year 2]
4. Measure how temperature influences the
performance of pIL nanolayers in selective sorption of CO2 over N2.
[proposed for Year 2]
5. Investigate selective transport of CO2
through membranes modified by pIL nanolayers. [newly added activity]
Research
progress
Our initial plan to examine the importance of
pIL nanolayer chemistry was to prepare ammonium-based IL monomers, and to use
these to grow pIL nanolayers. While the synthesis of the first monomer,
2-(methylacryloyloxy)ethyl-trimethylammonium tetrafluoroborate (1 in Figure 1), was successful, it
required a solution-phase purification step to isolate the product monomer.
After this first experiment, we redesigned our synthesis strategy to avoid the
monomer purification step. Rather than synthesize each monomer separately and
then prepare pIL nanolayers, we decided to use a single, commercially available
monomer, 2-(methylacryloyloxy)ethyl-trimethylammonium chloride (METAC) (2 in Figure 1), to prepare the polymer
nanolayers, and then carry out the ion exchange reaction on the grafted
polymer. A simple rinse step replaces the monomer purification step.
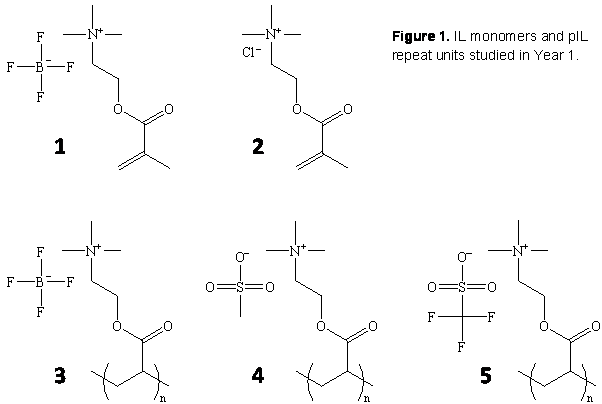
ATRP in a methanol/water solvent was used to
graft pMETAC from silicon wafers that had been coated with a 4-6 nm layer of
initiator-functionalized poly(glycidyl methacrylate). FTIR measurements
confirmed that polymerization was successful. pMETAC layer thickness evolution
was monitored by ex-situ ellipsometry. Figure 2 shows the evolution of layer
thickness with time for two catalyst formulations. As expected, increasing
Cu(II) concentration decreased growth rates and improved control (as indicated
by linear thickness evolution for open symbols).
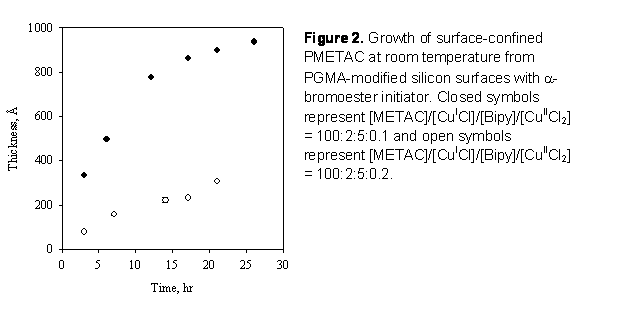
Following nanolayer growth, ion exchange was
done to replace Cl- with BF4-, CH3SO3-,
and CF3SO3-. To confirm that solid-phase ion
exchange was successful, we used XPS to determine atomic compositions of the
pIL nanolayers. Results verified that ion exchange reactions were quantitative.
No residual Cl was seen in any of the pIL nanolayers, and experimental atomic
ratios of F:N and S:N were similar to theoretical values.
Activities
3 and 4 were planned for Year 2; however, we were presented an opportunity to
do gas adsorption measurements using a Wicke-Kallenbach cell and analysis
methods developed by Prof. Seidel-Morgenstern at the Max Planck Institute for
Dynamic Complex Technical Systems,
Magdeburg, Germany. One student spent 3 months at the MPI measuring CO2
and N2 adsorption isotherms on pIL nanolayers grafted from cellulose
membranes. We used membrane supports instead of the proposed silica beads since
i) we envision pIL nanolayers to be used as CO2-selective layers for
membrane separations and ii) characterization of the pIL properties also can be
done on cellulose.
Figure 3 shows the adsorption isotherms at 25
�C for CO2 on pIL nanolayers comprising repeat units 3, 4,
5 (in Figure 1). There was no measureable N2 adsorption at the
highest pressure studied. Measurements were done at three temperatures. Data
analysis is under way to determine thermodynamic properties for adsorption. A journal manuscript is
being drafted to disseminate these results.
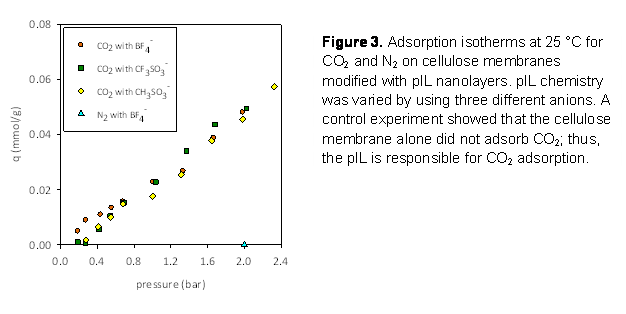
After demonstrating that the pIL nanolayers
adsorb CO2 selectively over N2, we investigated if a pIL
modified membrane would selectively transport CO2 over N2.
A pIL modified membrane was loaded into a home-built diffusion cell. The
downstream side of the cell was evacuated and the upstream side was pressurized
with CO2 or N2. Downstream pressure was monitored over
time. Figure 4a shows the results for an unmodified membrane. There is no
selectivity for either gas; both permeate through the membrane at the same
rate. Figure 4b shows the results for a membrane modified by a pIL nanolayer.
CO2 transport is much faster than N2 for this membrane.
Furthermore, the rate of pressure increase for CO2 is similar to the
unmodified membrane. These two results demonstrate that pIL nanolayers have
excellent potential for membrane-based CO2 separations.
Back to top