Back to Table of Contents
46271-AC4
The Oxygen Reactivity of Flavoenzymes: A Structural Approach
Andrea Mattevi, University of Pavia
Flavin-containing monooxygenases (FMOs) catalyze the
oxygenation of many nitrogen- sulphur-, phosphorous-, selenium-containing
nucleophilic compounds using molecular oxygen and NADPH as substrates (Figure 1).
These enzymes have become subject of intensive research because they are
involved in the metabolism of a wide range of drugs, carcinogens and other
xenobiotics.
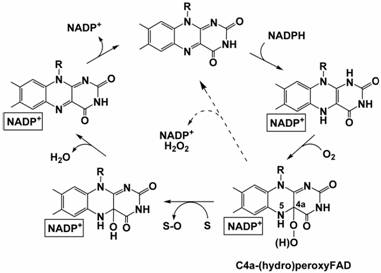
Figure 1. The catalytic cycle of
FMO.
The FMO catalytic cycle is complex and involves several
steps (Figure 1). The fundamental property of these enzymes is their ability to
stabilise the flavin-hydroperoxide. Stabilisation of the intermediate requires
the presence of NADP+, which remains tightly bound to the enzyme
throughout the catalytic cycle. Given these properties, it is evident that FMOs
represent an ideal system to study the structural enzymology of
flavin-hydroperoxide formation and, more generally, to gain insight into the
reaction of flavoproteins with oxygen.
Properties of Methylophaga FMO
A key aspect of mammalian FMOs biochemistry and
physiology is their association to the microsomal membranes through a
C-terminal hydrophobic segment acting as a membrane anchor. This has
complicated the heterologous over-expression of these proteins and their biochemical
and structural characterization. Recently, a bacterial FMO from Methylophaga
sp. strain Sk1 has been identified. This protein shares 31% sequence
identity and several functional properties with human FMOs. In the framework of
the ACS-PRF project, we have expressed and purified the protein which has
allowed us to confirm that the protein tightly binds FAD, reacts with
trimethylamine and similar nucleophiles, and, most importantly, appears to be
able to stabilise the flavin-hydroperoxide intermediate (Figure 2).
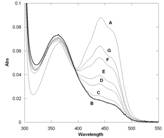
Figure 2. Absorbance spectra of bacterial FMO. Trace A represents the fully
oxidized form. Trace B was obtained by the aerobic addition of an equimolar
amount of NADPH and is consistent with a C4a-hydroperoxyflavin intermediate
containing a small amount of oxidized enzyme (shoulder at about 450 nm) due to
the intrinsic NADPH-oxidase activity. Traces C to G were sequentially recorded
after 25 sec, 90 sec, 3 min, 10 min, and 18 min and show the slow conversion of
the intermediate to the oxidized flavin.
Three-dimensional structure
We have solved the three-dimensional structure of
bacterial FMO at 2.7 Å resolution using seleno-substituted protein for solution
of the crystallographic phase problem. This result precisely represents the
main aim for the first year of the project as indicated in the original grant
application. The dimeric protein
is made up of two distinct domains, a larger FAD-binding domain and a smaller
NADP-binding domain. Both domains display a typical dinucleotide-binding fold
and are connected through a double linker that may act as a flexible hinge.
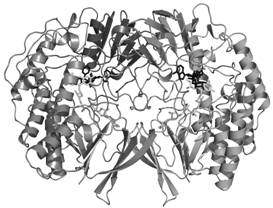
Figure 3. Overall three-dimensional structure of the Methylophaga FMO
dimer.
NADP+ binds in a cleft at the interface between
the two protein domains, blocking the access to the flavin and the catalytic
core. The nicotinamide ring is sandwiched between the re side of the
flavin and the single α-helix of the NADP-binding domain, and is roughly
coplanar with isoalloxazine at a distance of 3.0-4.0 Å. Most importantly, the
nicotinamide orients its reactive C4 atom away from the flavin N5 (C4-N5
distance of 5.1 Å) so that the NADP+ amide group is within
hydrogen-bond distance from the flavin O4 and N5 (Fig. 4).
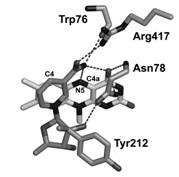
Figure 4. The active site of FMO.
Biochemical insight
Chemistry of NADPH requires that reduction of the
flavin cofactor is brought about through a hydride transfer from the C4 atom of
the nicotinamide ring to the flavin N5 atom. The stereochemistry observed in
the FMO crystal structure does not reveal a close proximity between these two
crucial atoms, and is clearly not compatible with a hydride transfer. This
implies that the structure in the crystals does not correspond to the
conformation in which NADPH is able to reduce the flavin. A modeling experiment
in which the hypothetical coordinates of the C4a-hydroperoxyflavin are
superimposed onto the flavin coordinates of the FMO structure places the two
additional oxygen atoms of the C4a-adduct within hydrogen-bond distance from
O2' atom of NADP+ ribose, which then would be perfectly positioned
to properly stabilize this oxygenating intermediate. These considerations suggest
that the conformation in FMO crystals is likely to correspond to that promoting
the stabilization of C4a-hydroperoxyflavin. Our structure is a clear
observation of a second fascinating functional property of NADP+ in
FMO catalytic cycle, i.e. the stabilization of C4a-hydroperoxyflavin.
NADP+ shields the active site and provides a proper H-bonding
environment that can prolong the intermediate half-life. Therefore, NADP+
is an integral part of the catalytic machinery that promotes the monooxygenation
reaction.
The scientific impact of these results is indicated by
the fact that they have been published in a high-profile journal such as PNAS.
This publication will also be important for the career of the two PhD students
(Andrea Alfieri and Roberto Orru) who are involved in the project.
Back to top