Back to Table of Contents
43351-G5
Investigation of Charge Transfer Processes via Electrochemical Separation of Stable Isotopes
Abby Kavner, University of California (Los Angeles)
The major goals of this research grant is to investigate chemical signatures of charge transfer processes under extreme conditions. We examined voltage-dependent separation of Fe and Zn stable isotopes during aqueous electrodeposition, showing that statistical thermodynamic model of charge transfer processes can predict stable isotope separation during electrochemistry. In a separate set of studies, we also examined how pressure affects the electrochemical behavior of the solid electrolytes AgI and AgBr. The stable isotope composition of transition metals can be used as tracers to elucidate the history of a variety of natural and man-made phenomena including ocean and solid Earth geochemical exchange, biological activity, and industrial processes. With the recent introduction and development of high-resolution mass spectrometry techniques, sub-part-per-thousand (�) level deviations from nominal isotope abundance can now be measured in many of these isotope systems, especially Zn, Fe, Cu, Cr, and Mo. In order to best use transition metal stable isotope markers to inform our understanding of Earth processes, and in order to exploit the isotope separation for industrial purposes, the physical and chemical processes underlying isotope fractionation must be quantified in the laboratory. In a first study, voltage-dependent amplification of stable isotope fractionation was observed for Fe electrodeposition. In a second study, we show a similar effect for a second transition metal, Zn. The results are interpreted using an extension of Marcus theory, predicting isotope fractionations as a function of driving force in an electrochemical system. Taken together with observations of natural fractionation of redox-sensitive and non redox-active elements, our modified Marcus theory provides a framework for quantitatively predicting transition metal isotope geochemical signatures during environmentally relevant redox processes in terms of simple energetic parameters. Isotope fractionation of electroplated Fe was measured as a function of applied electrochemical potential. As plating voltage was varied from 50 mV to 2.0 V, the isotopic signature of the electroplated iron became depleted in heavy Fe, with del56Fe values (relative to IRMM-14) ranging from -0.106(�0.01) to �2.290(�0.006) �, and corresponding del57Fe values of �0.145(�0.011) and �3.354(�0.019) �. This study demonstrates that there is a voltage-dependent isotope fractionation associated with the reduction of iron. We show that Marcus's theory for the kinetics of electron transfer can be extended to include the isotope effects of electron transfer, and that the extended theory accounts for the voltage dependence of Fe isotope fractionation. The magnitude of the electrochemically-induced fractionation is similar to that of Fe reduction by certain bacteria, suggesting that similar electrochemical processes may be responsible for biogeochemical Fe isotope effects. As with Fe, Zn metal is also enriched in the light isotope with respect to the Zn2+ leftover in solution, with a voltage-dependent fractionation factor. However, the slope of the fractionation vs. overpotential linear behavior has the opposite sign (See Figure). This can be interpreted, via a modified Marcus electrochemistry theory, as arising from differences in the equilibrium isotope fractionation factor between the products and the reactions in the Fe and Zn aqueous reactions. In addition to these studies of isotope fractionation versus voltage, in a third study, the experimental results are examined in the context of processes occurring at an electrode in an aqueous electroplating system, including charge transfer, mass transport, speciation, and temperature effects. We also performed an additional study involving miniaturization and pressurization of electrochemical devices. As mechanical devices become tinier, the need to explore potential micro- and nano- scale electronic devices becomes important. The goal of this seed project is to investigate the coupled electrochemical and mechanical behavior of the silver halides at extreme pressures in the range of 10^10 Pa, and at microscopic spatial scales in the diamond anvil cell. The silver halides have been shown to have superionic behavior--exceptionally high ionic conductivities in the solid state�at extreme conditions of mechanical stress and at elevated temperatures of ~100's of degrees. Preliminary experiments on the electrical and electrochemical behavior of AgI at high pressures in the diamond cell show a variety of interesting phenomena. The physical separation of silver and iodine phases from the AgI salt, whether due to a pressure gradient or an application of voltage, creates a chemical disequilibrium once the pressure or voltage source is removed. The stored chemical energy can be recovered only by a current flow accompanying re-association of the Ag and I2, implying that the AgI system is a miniaturized pressure-induced electrochemical device.
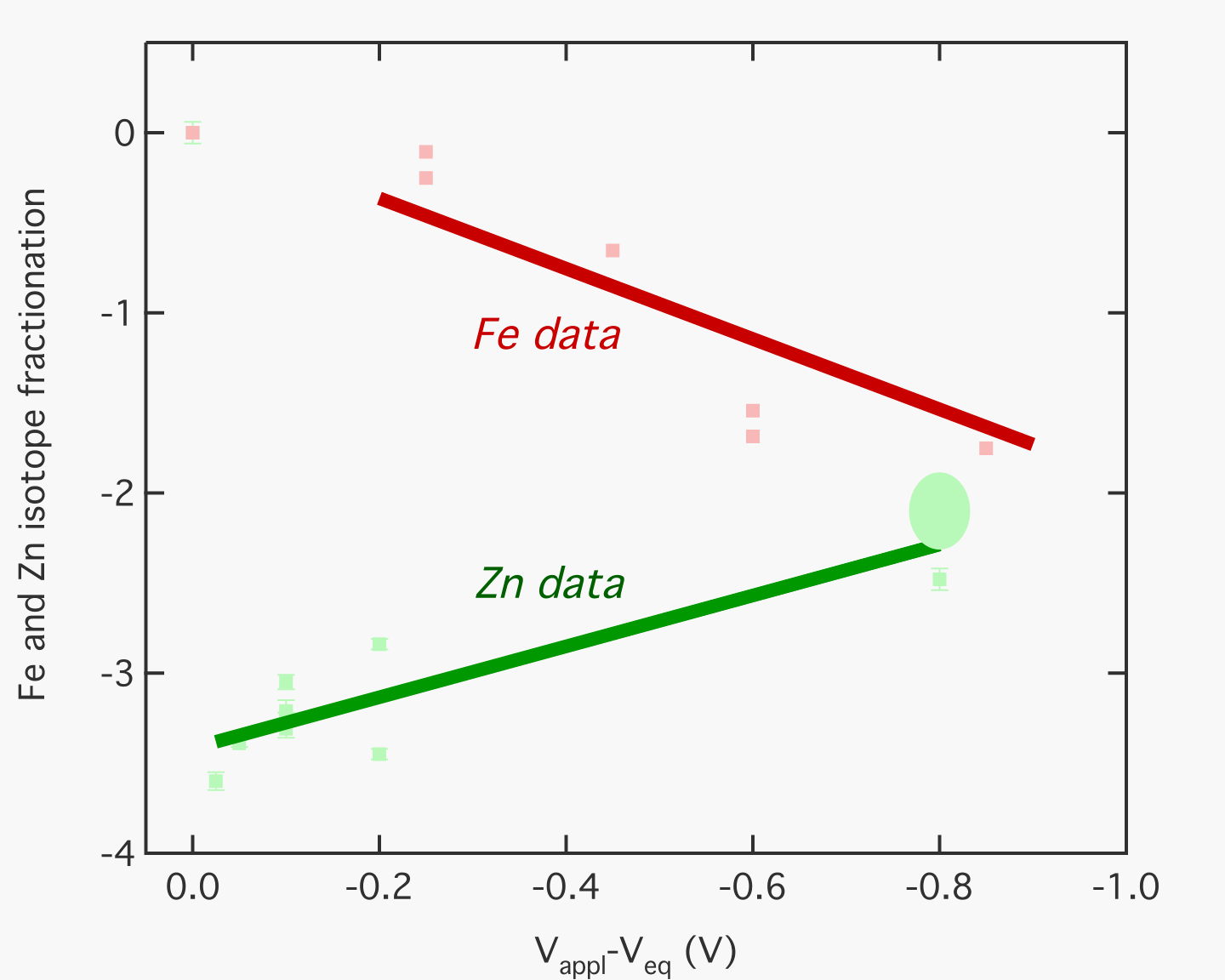
Back to top