Back to Table of Contents
41834-GB10
Topochemical Synthesis of New Layered Selenide Materials
Benjamin R. Martin, Texas State University - San Marcos
The proposed work centered on the synthesis of certain selenide precursor phases which possess structural features lending them amenable to further topochemical reactions. The ultimate goal of this work is the synthesis of kinetically stabilized phases that are not accessible via direct high temperature synthesis. In the past year we concentrated on ion exchange reactions using iodide salts. One of these experiments, detailed here, was a study of the ion exchange chemistry of the chain-like compound KFeS2 (Figure 1). This work was completed by two undergraduate students: Joseph Guy and Ross Spann.
KFeS2 is known to be isostructural to its rubidium analogue, RbFeS2. In 1976 Mossbauer spectroscopy was used by Nissen and Nagorny to study the solid solution KxRb1-xFeS2.[[1]] This was the first and only report of the existence of this solution, but no other characterization of the materials was conducted. We studied this material by directly synthesizing five members of this solution series, and by heating KFeS2 with RbI to generate mixed products by ion exchange.
The lattice constants of KxRb1-xFeS2 were found be linearly dependent on the substitution ratio, as predicted by Vegard's law (Figure 2a). When KFeS2 was heated with RbI in a 1:3 molar ratio to 520 oC for two hours, the product was found to be K0.27Rb0.73FeS2, which is very close to the predicted formula K0.25Rb0.75FeS2 for the cation mixture that was used. Interestingly, this exchange takes place rapidly in the solid state (the melting point of KI/RbI in this mixture is ca. 620 oC) Likewise, when KFeS2 was heated with RbI in a 1:1 molar ratio at 520 oC, the product was found to be K0.46Rb0.54FeS2, very close to K0.5Rb0.5FeS2. When the temperature was lowered to 450 oC over the same time period, a 1:3 molar ratio mixture of KFeS2 with RbI yielded K0.33Rb0.67FeS2. Finally at 400 oC and 350 oC the products of the exchange reactions were K0.44Rb0.56FeS2 and K0.63Rb0.37FeS2, respectively. At lower temperatures the exchange is incomplete due to the decrease in ion mobility. The lattice constants of the ion exchanged products closely match those of the compounds formed through direct synthesis (Figure 2a) with the exception of the compound formed at 350 oC. It is possible that the initial phase of the ion exchange proceeds inhomogeneously in a manner which distorts the a-axis, while the b-axis remains relatively unaffected.
This work was recently submitted to the Journal of Solid State Chemistry. Follow-up studies are planned using a high temperature powder X-ray diffraction chamber to study the kinetics of ion exchange from iodide salts. These results will help to clarify several previously published studies noting low temperature ion exchange reactions from iodide salts.
We are currently using similar alkali metal iodide exchange reactions to explore the phase space of other ternary sulfide and selenide compounds. Using these temperature variable methods we can observe products at different stages of exchange that help us to understand the kinetics of the exchange process.
Figure 1. KFeS2 unit cell viewed along the c-axis. Fe is located at the center of the shaded tetrahdra, sulfur is located at the corners of the tetrahedra, and potassium is represented by shaded spheres.
Figure 2. Plot of KxRb1-xFeS2 a- and b-axis lattice parameters as a function of potassium mole fraction (x). Data corresponding to the a-axis is plotted with open symbols (left axis), while b-axis data is plotted with filled symbols (right axis). (a) Compounds synthesized through the reaction of stoichiometric amounts of K2S2 and Rb2S2 with Fe and S. Linear regression yielded the two plotted lines. (b) Compounds synthesized through the reaction of a 1:3 molar ratio of KFeS2 with RbI at different temperatures. The lines are plotted following the equation solved from (a).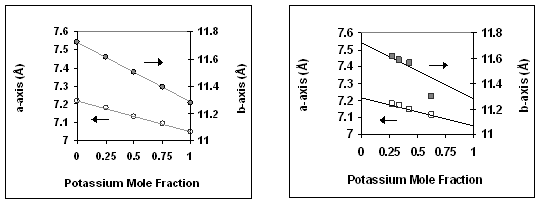
[[1]] Nissen, H. P.; Nagorn, K. Z. Phys. Chem., 99 (1976) 151-153.
Back to top