The advent of improved synthetic methods for metal nanoparticles has generated a widespread research effort on their optical, electronic, and chemical properties. Monolayer-protected metal clusters (MPCs) containing a few to a few hundred core atoms are of particular interest because they represent the bridge between bulk and molecular behavior. The core-size dependent electrochemical and optical properties of these small-core MPCs have been described.1 A significant challenge in this research is the development of synthetic methods capable of producing monodisperse nanoparticles in useful quantities with controlled size and shape for many applications in biological detection2 and catalysis.3
We have developed a facile preparative route to alkanethiolate (Cn) Au38 nanoparticles, where n = 4, 6, 8, 10, and 12, that could serve as a model system for investigating the interparticle electron transfer dynamics. Subnanometer-sized nanoparticles are initially produced by a modified Brust synthesis,4 which undergo core-size evolution upon removal of reaction impurities that have served as additional protecting layers.
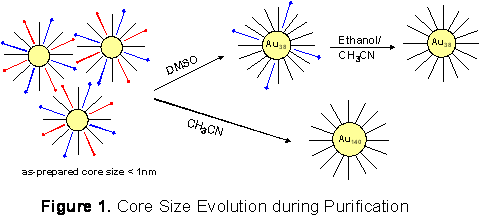
Figure 1 illustrates the proposed core-size evolution mechanism. The as-prepared MPCs (core diameter <1nm) are stabilized in the presence of the reaction impurities (red and blue). Selective removal of charged Oct4N+ (red) yields stable Au38 MPCs that maintain their core size during the following cleaning process with ethanol and acetonitrile, whereas extensive� removal of the reaction impurities by acetonitrile produces rather polydisperse larger Au140-like MPCs. Organothiolate-coated MPCs are known to be very stable even in the dried state.5 The results presented here suggest, however, that the stability may be greatly diminished for very small-cores that only exist in the presence of excess protecting layers including reaction impurities. When these impurities are removed, small-core MPCs tend to aggregate to form more stable Au38 or larger Au140 MPCs.
����������������������� 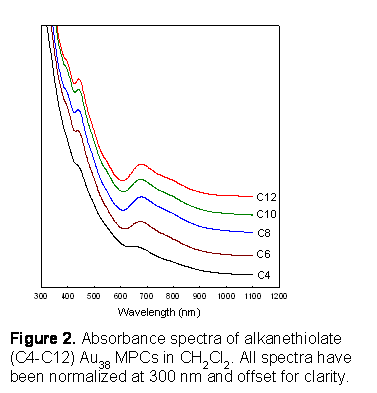
����������� The proposed preparative route (Figure 1) to C6-Au38 MPCs was examined for other alkanethiol ligands. The TEM results of C4-C12 Au38 MPCs show that the cores are relatively monodisperse with average core diameter of 1.1-1.2 nm, indicating the preparative method for the Au38 MPCs works for all alkanethiolates from C4 to C12. The formation of Au38 MPCs were also confirmed by absorbance spectra which all displayed (Figure 2) the characteristics of Au38 MPCs,6 i.e., distinct peaks at 670, 460, and 400 nm are indicative of molecule-like electronic levels of Au38 MPCs. The synthetic yield of C4-Au38 MPC was 7.8 % based on moles of the gold reactant. The yields increased to 17-24 % with longer chain ligands (C6-C12), implying longer ligands more effectively prevent core aggregation and thus favor Au38 formation.
����������������������� 
����������� �����������������
����������� Figure 3a shows 25 �C DPVs for CH2Cl2 solutions of Cn-Au38 MPCs, where n = 4, 6, 8, 10, and 12. Again, all DPVs exhibit the characteristics of Au38 nanoparticles; the patterns of current peaks are very similar to one another, consisting of a large central gap between the first oxidation (ox1, ca. -0.65 V vs. Fc/Fc+) and the first reduction step (re1) and a second (ox2) and third oxidation (ox3) step spaced by, respectively, a small and a larger potential interval. The first two oxidation peaks (ox1 and ox2) are chemically reversible on the voltammetric time scale employed in Figure 3a for shorter ligands (C4 and C6) but become irreversible for longer ligands, displaying symptoms of product instability. The reversibility is improved at lowered temperature (-78 �C, Figure 3b) where the ox2 reaction becomes reversible for longer ligands and the ox3 reaction becomes nearly so. In addition, there are more reduction steps observed at lowered temperature. We were unable to obtain a resolved DPV for the C12 Au38 MPC presumably due to its low solubility in CH2Cl2 at lowered temperature. The voltammograms in Figure 3a and b display a high ratio of peak currents to background currents, meaning that the MPC samples are reasonably monodisperse in the electrochemical context.
����������� In summary, the voltammetric peak patterns of these Au38 MPCs display a large, ligand-independent electrochemical HOMO-LUMO gap (ox1-re1) and ligand thickness dependent ox2-ox1 and ox3-ox2 potential spacings, both of which increase with the ligand thickness. These voltammetric results indicate that the charging energetics of Au38 MPCs is characterized by a combination of the discrete core electronic levels and surrounding dielectric medium.
1. S. Chen et al. Science 1998, 280, 2098.
2. R. Elghanian et al. Science 1997, 277, 1078.
3. T. S. Ahmadi et al. Science 1996, 272, 1924.
4. M. Brust et al. J. Chem. Soc., Chem. Commun. 1994, 801.
5. A. C. Templeton et al. Acc. Chem. Res. 2000, 33, 27.
6. D. Lee et al. J. Am. Chem. Soc. 2004, 126, 6193.