Reports: AC10
47578-AC10 Organic Semiconductor Blends Designed for High Seebeck Coefficient through Fermi Level and Density of States Engineering
Howard E. Katz, Johns Hopkins University
Figure 1 .Schematic of proposed organic thermoelectric composite, consisting of an additive that sets the Fermi level and a bulk in which charge carriers at higher energy levels are transported.
|
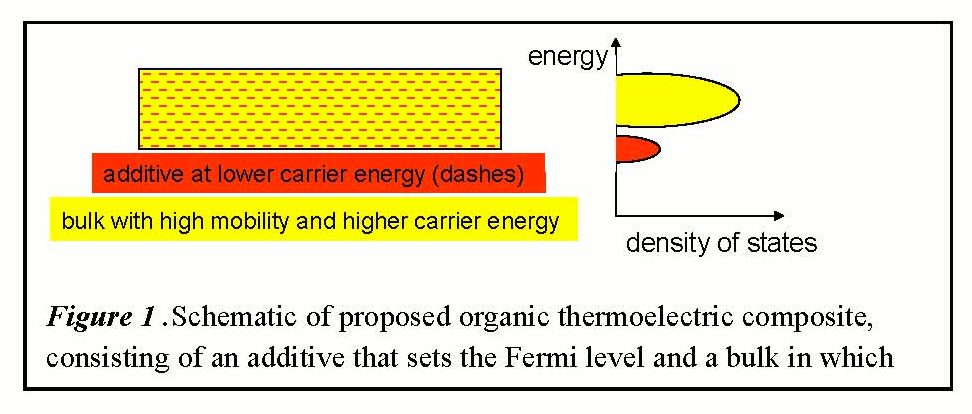
This project has produced initial results on
semiconductor blends in which the major component, bulk molecular subunits,
are designed to have carrier energies just above orbital energies of a minor
component additive. The Fermi level is
established by the additive, while the current from injected charge is carried
predominantly in the higher energy orbitals of the bulk, as shown in Figure 1.
The additive
is not a dopant; in fact, we add a dopant as a third component to increase
conductivity. Doping would not greatly
alter the Fermi level, in contrast to previously described one-compoent systems
considered for thermoelectric applications.
This situation is effectively equivalent to having a large derivative of
density of states with respect to energy.
In cooling mode, injected charge transfers energy across a sample. In powering mode, the thermal excitation of
additive-centered charge carriers in one region of the sample will lead to the
migration of some of them into the bulk energy levels of another region of the
sample, producing a thermovoltaic effect.
The significant accomplishment is
that unlike in any previous studies, a regime is identified in which Seebeck
coefficient and electrical conductivity both increase as a result of
doping. This phenomenon is not observed
in controls without the additive in which charge carriers are more stable.
Figure 6. Plots of S and conductivity versus sample number for each data set, 1-4, clockwise from top left.
|
Seebeck coefficients and
conductivities. Data from films containing mixtures of
poly(hexylthiophene), poly(hexylthiothiophene) as the minor, lower hole-energy
additive, and tetrafluorotetracyanoquinodimethane dopant were obtained. Each Seebeck coefficient is the slope of a
plot of measured voltages versus temperature difference. For the blends with increased amounts of
P3HTT (sets 3 and 4, Figure 2), there are regimes in which Seebeck coefficient
and electrical conductivity increase simultaneously. Figure 2 shows plots of Seebeck coefficient
and electrical conductivity as a function of doping level for each data
set. While the highest thermopower was
actually obtained in set 2, the most interesting scientific outcome is the
trend of sets 3 and 4. Note that for the
first three or four data points in these sets, S and conductivity both increase
as doping level increases. To our
knowledge, this is the first report of the maintenance or increase of S as
doping is increased. Even for set 2,
the blending of the two polymers prevents or diminishes the generally observed
decrease in S as dopant is added.
The project was
the vehicle to introduce thermoelectricity to my research group of five
graduate students, five postdoctoral fellows, and three undergraduates, as well
as myself, the PI. Four group members
became well versed in the theory of thermoelectricity and the practice of
performing the experiments. One graduate student will have thermoelectricity as
a main theme of a Ph.D. thesis, and another student will apply the principles
to a thesis on related topics. Thermoelectricity
is becoming a tool for our analyses of other electrical phenomena in organic
materials, including charge separation in photovoltaics and charge injection
barriers in organic transistors. The
project also opened a major collaboration with a world-leading group studying
thermoelectrics at the University of California and provided exposure to
organic materials to mechanical engineering students there. It would not have been possible to achieve
these intellectual advances through any other agency because of its being a
departure from activities that had been ongoing in the research group before.