Back to Table of Contents
44942-AC3
Characterization of Catalytic Imide Group Transfer Reactions with Iron Catalysts
Patrick L. Holland, University of Rochester
Since the submission of this proposal, we have published the results described therein as preliminary information (N. A. Eckert, S. Vaddadi, S. Stoian, C. J. Flaschenriem, T. R. Cundari, P. L. Holland, "Coordination Number Dependence of Reactivity in an Imidoiron(III) Complex," Angew. Chem., Int. Ed. Engl. 2006, 45, 6868-6871). The 2007 progress report relates the chemistry accomplished since then.
(1) Characterization of Iron(III) Imido Complexes. The imido complex we have reported is shown at the left of Figure 1, but it is too unstable for crystallography, and is formed in less than 70% yield, hindering analysis by EXAFS (which would aim to show the presence of a short Fe=N bond). We aim to create more stable imido intermediates for crystallography and/or EXAFS analysis, through ligand variation (Figure 1). First, adding tert-butyl groups to the backbone adds steric hindrance (Figure 1, center). Using this ligand, we have generated solutions that contain 85-90% imido species, as judged by 1H NMR spectroscopy. These solutions are sufficiently pure for use of EXAFS and Mšössbauer spectroscopy to give more detail into electronic structure.

Another ligand modification is shown at the right of Figure 1. This ligand has no weak C-H bonds near the metal site, because the ortho-isopropyl groups are replaced by tert-butyl groups. We have demonstrated the coordination of this ligand to iron, and are trying to create its imido complexes.
(2) Hydrogen Atom Abstraction and Amination Reactions. This section of the proposed work is dependent on part (1), demonstrating a ligand that is not attacked by the imido group. We will address intramolecular H-atom abstractions in the coming year.
(3) Catalytic Carbodiimide and Aziridine Formation through Group Transfer. We have nearly finished our mechanistic studies on the catalytic formation of tBuNCNAd from adamantyl azide (AdN3) and t-butyl isocyanide (tBuNC). The rate law of the reaction is rate = [Fe][AdN3][tBuNC]-2. EPR studies on catalytic reactions flash frozen during catalysis show that it is identical to a solution generated from adding excess isocyanide to an iron(I) dinitrogen complex. The axial EPR signal (g = 2.09, 2.07, 2.00) is assigned as LtBuFe(CNtBu)3 by the similarity of this signal to that for LMeFe(CO)3, previously characterized in our group. Therefore, under catalytic conditions, the major compound present in solution is LtBuFe(CNtBu)3, the resting state. Combining this with the rate law suggests the mechanism shown in Figure 2.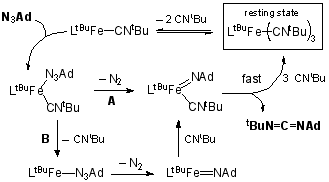
Finally, we have tested a range of azides and isocyanides. Adamantylazide has been the only capable nitrene fragment, and a range of isocyanides undergo catalytic carbodiimide formation. We have fully characterized AdN=C=NtBu and AdN=C=NCy.
(4) Other Results. During attempted syntheses of LMeFe=NAd, we have isolated two interesting species: a tetrazene complex LMeFe(AdNNNNAd), and a "hexazene" complex (LMeFe)2(AdNNNNNNAd). Each contains a very interesting polynitrogen ligand, and each has been evaluated using X-ray crystallography. The "hexazene" group (AdNNNNNNAd) is exciting because it is previously unknown in transition-metal chemistry. The tetrazene complex is interesting for a different reason: it could be formulated as an iron(I) complex with a neutral ligand, as an iron(II) complex with a radical anion ligand, or an iron(III) complex with a dianionic ligand (Figure 3). Any of these could be consistent with the S = 3/2 ground state seen in magnetic studies. Interestingly, Möšssbauer spectra are most consistent with the iron(II) formulation. If confirmed by EPR, this would demonstrate the first example of a tetrazenyl radical ligand. We anticipate publication of these interesting tangents soon, while continuing to place primary focus on stabilizing imido complexes for use in amination and aziridination reactions.

Back to top