Back to Table of Contents
41155-B10,4
Molecular Topology Directed Crystalline Architectures
Kraig A. Wheeler, Eastern Illinois University
The first likely occurence of quasiracemates in the literature dates back more than one hundred and fifty years to Pasteur's celebrated tartaric acid work. In addition to providing extensive experimental details of the recrystallization outcomes for tartaric acids (e.g. growth conditions, solubility, crystal face measurements, and optical properties), L. Pasteur described the formation of unusual compounds he referred to as combination isomers. The two examples cited in his 1853 paper were derived from ammonium (+)-bitartrate/(-)-bimalate and (+)-tartramide/(-)-malamide components. These materials showed diminished optical bevaviour and notably different solubilities than the enantiopure building-blocks. An additional curiosity resulting from this work includes the crystal morphologies of the ammonium salt. Pasteur's account of this material draws attention to the intergrowth of three distinct crystals. The central portion was assigned as ammonium (+)-bitartrate and the outer two crystals as the combination isomer. These results raise several important questions such as are these combination isomers quasiracemic compounds and what structural features are responsible for this peculiar crystal morphology?

Fig 1 Photograph (left) and sketch (right) of three-component crystals formed from recrystallization of ammonium (2R,3R)-(+)-bitartrate and ammonium (S)-(-)-bimalate
Given the historical significance of Pasteur's work and potential application to quasiracemates, we set out in this grant period to explore and crystallographically assess his combination isomers. Following Pasteur's procedure, we were able to prepare crystalline samples that resemble the description in his 1853 account. These transparent colorless samples consist of unusual, but uniform crystal morphologies that appear to be derived from the deliberate combination of three distinct crystals (Fig. 1).
The three-component crystals were separated and full single-crystal X-ray diffraction studies performed on each fragment. Assessment of these samples confirmed Pasteur's findings that the central block consists of ammonium (+)-bitartate and the lathes as quasiracemates containing ammonium (+)-bitartate/(-)-bimalate. Each section of the three-component crystals align with the c–axis as the contact direction.

Fig. 2 Crystal structure of the quasiracemate ammonium (+)-bitartrate/(-)-bimalate dimer showing approximate inversion symmetry.
As shown in Fig. 2, the crystal structure of the lathes reveals ammonium cations and equal portions of (2R,3R)-(+)-hydrogen tartrate and (S)-(-)-hydrogen malate anions assembled via N+-H···O to give dimeric motifs. Although the tartrate and malate components are chemically distinct, this collection forms approximate inversion relationships in space group P21. The use of all hydrogen-bond donor (NH and OH) and acceptor (O atoms) groups creates a intricate network of noncovalent interactions. Each tartrate and malate component participates in strong CO2H···-O2C interactions with translationally related neighbors to give homomeric 1D chains along the a axis.
The structure of the central ammonium (+)-bitartate crystal corresponds to a known orthorhombic phase (space group P212121). Each tartrate moiety forms homomeric molecular strands that are linked by ammonium cations. Although the overall topology of each strand and the position of the ammonium N atoms are similar to that observed for the quasiracemate, several notable variations exist. The most striking difference is the parallel alignment of tartrate strands. These strands are connected by NH4+···-O2C/HO2C motifs that give rise to hydrogen bond contacts that differ markedly than the hydrogen bonded dimers observed for the quasiracemate.
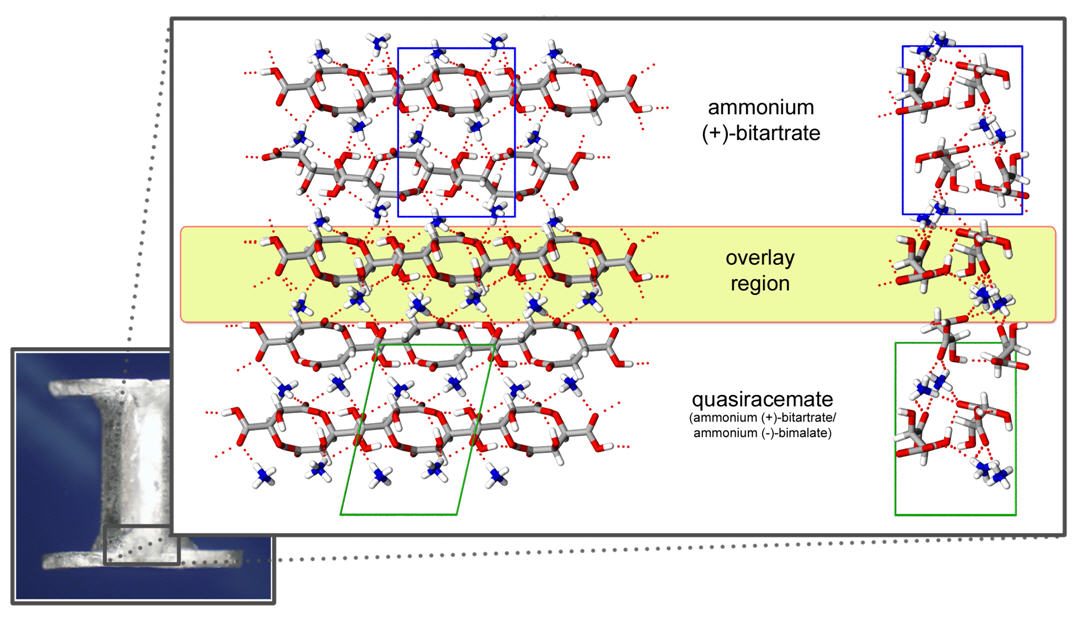
Fig. 3 Crystal structure projections showing the unit cells and overlay at the crystal interface of the (a) quasiracemate and (b) ammonium (+)-bitartrate components.
Spurred by the curisousity of this face-specific intergrowth phenomenon and the diversity of crystal packing of the component phases, we set out to explore the structural relationships at the crystal interfaces. Fig. 3 depicts the overlay of the quasiracemate and ammonium (+)-bitartate crystal structures. The overall crystal packing of these phases is remarkably similar despite the variation in crystal systems, unit cell parameters, and directionality of catemeric motifs. Careful inspection of the interface region of the quasiracemate and (+)-bitartrate phases reveals that the alignment of crystal faces coincides with a near perfect overlap of ammonium (+)-bitartrate strands. The NH4+ molecules provide the most discernable, albeit subtle, structural difference with only a slight variation in H atom positions.
This study has provided important insight into Pastuer's quasiracemates and contributes to the current understanding the processes of molecular recognition. The combination of quasiracemate discovery and epitaxial growth of these three-component crystals has given the PI and student researchers at Eastern Illinois University new areas to explore and assess the structural preferences and utility of quasiracemic materials.
Back to top