Back to Table of Contents
43240-AC3
Application of s-Block Molecular Aggregates in the Controlled Assembly of Solid-State Materials
Kenneth W. Henderson, University of Notre Dame
Our project has been directed towards the incorporation of s-block metals in metal-organic frameworks (MOFs) and coordination polymers. The area of MOFs has received considerable attention in recent years due to the potential of the materials prepared in applications such as catalysis, optics, electronics, small molecule storage, and separation science. A popular strategy for the synthesis of these networks has been through the combination of transition metal salts with ditopic carboxylate ligands. In comparison, relatively little attention has been given to the use of s-block metals in the construction of MOFs. However, the use of such metals in porous materials is appealing as they are predicted to have strong binding affinities for some small molecules such as dihydrogen, making them candidates as lightweight sorption materials. Furthermore, considering the widespread use of s-block metal complexes in both organic and in inorganic synthesis, the preparation of porous solids incorporating these elements opens up the opportunity to create solid-state reagents. During this project we and others have prepared the first examples of permanently porous magnesium carboxylate frameworks. These materials have proven to be more thermally robust than their transition metal analogues, and have been shown to be useful in the molecular exchange of small molecules and also in the sorption of various gases including dihydrogen. We recently extended our work in this area towards the formation of homochiral frameworks for use in enantioselective separations and heterogeneous asymmetric syntheses. An example of a particularly interesting chiral framework material is shown in Figure 1. The structure of [Mg2(Hcam)3×3H2O]×NO3, 1, (where H2cam = (+)-camphoric acid) consists of a series of fused Mg12 cages which have twelve water molecules at their centers, creating isolated 0D cavities within the structure. Overall, the extended structure is a body-centered cubic (bcu) lattice, with the Mg12 cages being utilized as eight-connected nodes.

Figure 1. X-ray structure of 1 showing the repeating Mg12 cage building block with the red sphere depicting the 0D space occupied by water.
Furthermore, we have used this system to investigate the use of electrospray ionization mass spectroscopy (ESI-MS) as a tool to study the assembly of MOFs. This has proved to be successful, with the fundamental building blocks, three-fold [Mg2(Hcam)3]+ paddle wheels, being identified in solution. This work suggests that ESI-MS may be a very useful technique in rationalizing or even predicting the structures of complex MOF materials by the analysis of precursor solution species.
Another area of active study has been constructing extended polymers through the use of directional cation-p interactions. This is a novel method for the synthesis polymers and one which is particularly suitable for heavy s-block elements. We have shown that ferrocene can be used as a linear ditopic linker in association with alkali metal aggregates to form coordination networks. A particularly significant series of compounds arising from this work is the homologous set of complexes [{(Me3Si)2NM}2·(Cp2Fe)]¥ (where M = Na, K, Rb or Cs). All of these complexes form linear polymer with ferrocene bridging between M2N2 dimers. Figure 2 shows the extended structure of the rubidium complex which illustrates the network formed through a combination of cation-p and agostic interactions.
Figure 2. Section of the extended structure of [{(Me3Si)2NRb}2·(Cp2Fe)]¥.
This work demonstrates that cation-p interactions can be used to assemble polymers in a directed fashion. This opens up the possibility of preparing interesting magnetic or optical functional materials using this strategy.
Finally, we have recently discovered that new aggregated forms of magnesium imides, RNMg, may be prepared using halide-substituted anilides. Specifically, the first examples of magnesium imide Mg4N4 cubanes have been prepared using 2,4,6-trichloroanilide as ligand (Figure 3). Previously, only hexameric structures were known and this work suggests that a rich variety of aggregated structures are available through the use of appropriate types of ligands.
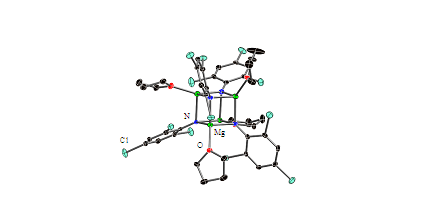
Figure 3. Molecular structure of [2,4,6-Cl3C6H2NMg·diox)4.
Back to top