Reports: G9
47731-G9 Effect of External Flow Fields on Self-Assembly of Colloidal Suspensions: Modeling and Experiments
This project seeks to examine the effect of flow field on self-assembly of colloidal suspensions via combined modeling and experiments. In the reporting period, the primary focus is on the wetting, evaporatively driven flow, and resulting particle deposition of inkjet-printed colloidal drops on various substrates.
In the modeling effort, a two-dimensional mass conserving lattice Boltzmann method (LBM) has been developed for multiphase (liquid and vapor) flows with solid particles suspended within the liquid and/or vapor phases. The main modification to previous single-phase particle suspension models is the addition of surface (adhesive) forces between the suspended particle and the surrounding fluid. The multiphase dynamics between fluid phases is simulated via the single-component multiphase model of Shan and Chen [1]. The combined multiphase particle suspension model is first validated and then used to simulate the dynamics of a single-suspended particle on a planar liquid-vapor interface and the interaction between a single particle and a free-standing liquid drop. It is observed that the dynamics of suspended particles near free-standing liquid droplets is affected by spurious velocity currents although the liquid-vapor interface itself is a local energy minimum for particles. Finally, results are obtained for capillary interactions between two suspended particles on a liquid-vapor interface subjected to different external forces and for spinodal decomposition of a liquid vapor mixture in the presence of suspended particles. Qualitative agreements are reached when compared with results of suspended particles in a binary mixture based on multi-component LBM models.
To model the surface tension gradient driven flow induced in an evaporating drop, a two-dimensional (2D) LBM recently developed by Chibbaro et al. [2] for simulating single component two-phase (liquid and vapor) flows with independent control of the interface tension between the liquid and vapor phases is extended to include solid walls and the associated adhesive forces. The interface tension is coupled to the temperature via a passive advection-diffusion thermal LBM model. The coupled flow and thermal LBM is used to study thermo-capillary flows inside a liquid drop on a substrate, where the drop is in equilibrium with its vapor phase and has attained an equilibrium contact angle with respect to the substrate. Marangoni flow patterns from the LBM simulations show good qualitative agreement with prior numerical simulations and experiments. Figure 1 shows the steady-state interface profile and velocity field in the symmetrical right half of the drop for different ds/dT values. As expected, liquid near the interface moves from regions of low interface tension (near the substrate) to regions of high interface tension (towards the apex of the drop). Because mass has to be conserved, this causes two opposite convective rolls to form inside the drop at steady state, driving fluid towards the substrate near the center of the drop.
On the experimental side, an experimental investigation has been carried out to examine the deposition dynamics of inkjet-printed colloidal drops on glass and PET substrates. Using fluorescent particles and confocal microscopy, the evaporatively-driven and thermal Marangoni flows inside a colloidal drop, as well as the particle self-assembly and deposition on the substrate are directly observed. Figure 2 shows a sequence of frames recorded from the impact and subsequent evaporation of a single jetted drop on an acetone cleaned glass substrate. The first four frames clearly show a continuous and radial flow of the particles away from a pinned contact line and towards what appears to be the center of the drop. This flow continues until a certain minimum radius of the particle group is attained (frame 4). After a momentary pause at this state of minimum grouped size, the particles begin a reversed radial flow towards the contact line. As the particles reach the contact line, they adhere to the surface of the substrate in a coffee-ring pattern (frame 7). At some time in the progression of this flow, the contact line begins to de-pin in a stick-slip manner until the majority of the solvent has evaporated and only the particle deposition pattern remains (frame 8). Ar plasma treatment is used next to modify the substrate surfaces. Wetting properties (i.e., advancing /receding contact angle and contact angle hysteresis) of colloidal drops on different substrates, substrate surface roughness, and final particle deposition morphologies are characterized using goniometer, AFM, and SEM. A correlation between the substrate surface roughness, static receding contact angle, contact line pinning/de-wetting, and final particle deposition morphology is explored.
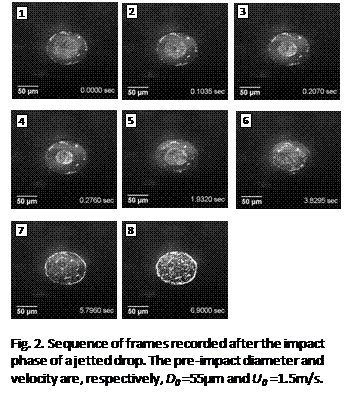
Using fluorescence microscopy, the effect of particle size on deposition dynamics of inkjet-printed colloidal drops is studied with monodispersed polystyrene particles in the size range of 0.02 to 1.1 µm on glass, Ar plasma cleaned glass, and PDMS coated glass substrates. The results show that the substrate properties play an important role in determining the final dried patterns formed by the colloidal particles. Our observations also reveal that particle size and contact angle formed by the solvent in the dispersion determine how close to the contact line the particles can be deposited. It is found that smaller particles can move closer to the deposited contact line than particles with bigger sizes.
Future work will focus on the contact angle hysteresis and the stick-slip behavior of the contact line motion as a colloidal drop wetting and evaporating on a patterned substrate using three-dimensional model simulations. An experimental investigation will be carried out to explore the use of organic solvents and its effect on evaporation and Marangoni flow of a colloidal drop.
References:
[1] X. Shan and H. Chen, Phys. Rev. E 47, 1815 (1993).
[2] S. Chibbaro, G. Falucci, G. Chiatti, H. Chen, X. Shan, and S. Succi, Phys. Rev. E 77, 036705 (2008).