Reports: G7
47000-G7 Microrheological Investigation of Particle-Laden Polymer/Polymer Interfaces
Microrheological Investigation of Particle-Laden Polymer/Polymer Interfaces
The goal of our PRF-G project is to measure the interfacial rheology of polymer-polymer interfaces that contain various surface-active species – examples include nanoparticles, colloids, and block copolymers. Last year, our report focused on the use of a novel technique to measure the interfacial rheology of various model systems. This year, our report focuses on two aspects related to this project: (1) We have continued to employ our interfacial microrheometer, and have specifically measured the viscoelastic moduli and history-dependence of a phospholipid monolayer at the air/water interface, and (2) we have initiated studies into polymer/polymer interfaces, and have worked to identify and deal with the new issues that arise there with respect to interfacial preparaction and characterization, as well as the different scales for measurement.
Interfacial microrheometry of phospholipid monolayers.
Our group has developed an interfacial microrheometry system, in which we microfabricate amphiphilic, ferromagnetic disks that serve as microrheological probes with controllable surface chemistry and geometry. Using electromagnets to exert a (known) torque upon the disk, while simultaneously measuring the disk's orientation, gives the interfacial visco-elastic moduli, so long as the rotational drag force upon the disk is dominated by the interface. The small scale (~10 micron) of the probe naturally make it exquisitely sensitive to interfacial viscoelasticity, relative to the bulk, and furthermore enables measurements with smaller sample volumes.
Fig. 1. Soft, glassy rheology of DPPC in the liquid-condensed phase. Blue squares: surface elastic modulus, which exhibits a low-frequency plateau that dominates the material behavior. Higher-frequency behavior is dominated by the viscous response (triangles). Close-packed LC grains can not readily rearrange, giving the low-frequency elastic plateau. |
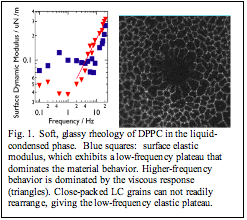
We have worked with the phospholipid Dipalmitoyl
Phosphatidicholene (DPPC) as a model monolayer, motivated by scientific
interest, by the predominant role DPPC plays in the lung surfactant system, and
by techniques that had been developed for its visualization. Specifically, we measured the
frequency-dependent, linear viscoelastic surface shear moduli of DPPC at lower
surface pressures than have previously been possible. (Almost all previous measurements of DPPC concerned the
steady shear viscosity.) We found
the interfacial rheology of DPPC in the weak liquid-condensed (LC) phase
(surface pressure Ps ~ 8-10
mN/m) to be consistent with that of a soft glassy material: elastic at low frequencies, and viscous
at high frequencies. This stands
in contrast with most viscoelastic soft materials, which are viscous at low
frequencies (as microstructures relax) and elastic at frequencies high enough
that the structures can not. Soft,
glassy materials are both soft and glassy: they can be deformed, and yet can be kinematically trapped
in long-lived metastable states.
Direct visualization of the DPPC monolayer using a small fraction of
fluorescently-dyed lipid, which naturally segregates to LC domain boundaries,
shows the microstructural origin of this behavior: LC grains are themselves elastic, and can not readily slide
past each other without wholesale rearrangement of many neighboring
grains. (Figure 1) Present efforts are directed towards
measuring and understanding the history-dependence, ageing and yielding of the rheological response of DPPC, as well as adapting the
microdisk rheometer to much more viscous polymer/polymer systems.
Interfacial microtensiometry
In adapting our methods to the polymer/polymer systems that originally motivated our proposal, we experienced difficulties in characterizing surfactant-laden polymer/polymer systems. In particular, the Wilhelmy plate that is routinely used to measure the surface pressure of Langmuir monolayers at liquid/air interfaces becomes difficult or impossible to use for the small-scale polymer/polymer interfaces of interest here. Under separate funding, we developed a microscale method for interfacial tensiometry, in which we used microfabrication techniques to make small elastic structures that isolate one portion of the interface from another, and which deform in response to differences in surface pressure between the two. By measuring the deflection of the micro-tensiometer arms, and using elastic beam theory to determine the surface pressure difference between inside (which we prepare without surfactant) and outside (which generally contains the surfactant of interest), we effectively measure the surface pressure of the outside.
With PRF-G support, we have employed this micro-tensiometer to measure and characterize the surface pressure-concentration isotherm of DPPC, with which we now have extensive experience. The agreement is excellent. We are now working to adapt this to polymer-polymer systems, which will require geometric modifications (in order to be sensitive to the much lower surface pressure ranges expected for polymer-polymer systems), and perhaps a change of microtensiometer material (from a photoresist polymer to a more conventional molecular solid like Silicon or Silica), to prevent solvent from swelling the device. We are also working to develop expertise and protocols for reliably preparing and measuring surfactant-laden polymer-polymer interfaces, for which conventional techniques developed for air/water interfaces are at times ineffective or prohibitively slow.
Figure 2. Microfabricated, freely-floating tensiometers (inset) segregate the internal interface (surfactant-free) from the external interface (surfactant-laden). Compressing the outer interface changes the external surface pressure and deflects the arms of the tensiometer to a degree which can be predicted using elastic beam theory. Micro-tensiometer measurements (open circles) agree quantitatively with the standard Wilhelmy plate (red line) for a DPPC monolayer. |
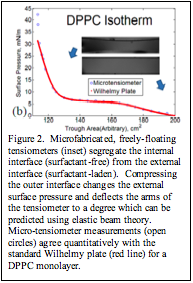