Reports: B6
44760-B6 Thermodynamics and Kinetic Studies of Hydrogen Isotope Binding on Selective Materials
Selective hydrogen isotope binding
Our studies on the preferred tritiated water auto-dissociation confirm that T+ is the preferred target over TO-. With this in mind, we have started developing a model for hydrogen isotope conduction through a membrane channel.
I. The tritiated water auto-dissociation equilibria: As a first step toward understanding the thermodynamics and kinetics of tritium exchange in a variety of materials, we considered the tritiated water auto-dissociation equilibria. Identifying the preferred form of the tritium (T+ or TO-) is key to choosing materials that can exchange it. To find the change in free energy of each of these reactions, we found the solvation free energy of the individual species using a quasi-chemical theory of solvation.[1] Lindiwe Ndebele '09 and Laura Fernandez Ô08 have found the most probable solvated structures for T+ and TO- using a quasi-chemical theory of solvation with harmonic and anharmonic frequency estimates. The most probable species using harmonic frequency estimates are the same as those found in previous quasi-chemical applications on H+ and HO-.
Since differences between isotopes are crucial for the auto-dissociation of tritiated water, we have considered anharmonic frequencies. A second order perturbation approach yielded highly suspect frequencies for the highly anharmonic hydrated hydroxide species so we turned our attention to simple scaling relations. At room temperature, we find a pKw of 14 for H2O and both possible HTO dissociation harmonically. Including scaling relations to anharmonic behavior changes the pKw of HTO dissociation into HO- and T+ to 11 while leaving the other dissociations at 14. Since experimental studies suggest that raising the temperature from 20°C to 60°C increases the HTO dissociation preference towards HO- and T+, we are considered the same equilibria at 60°C. At this elevated temperature, we found that the preferred number of waters solvating hydroxide shifted to smaller numbers. The pKw for water dissociation also decreased to 12 and 9 using the harmonic and anharmonic approximations, respectively. The direction of the shift agrees with water experiments but the magnitude of the shift is too high. The CRC value for water pKw is 13 at 60°C. The shift is already wide in the harmonic case and too wide anharmonically suggesting that rescaling of frequencies is not sufficient to capture anharmonicities accurately. Using the harmonic approximation the pKw for HTO dissociation into either set of species is 12. The anharmonic pKw for tritiated water dissociation into HO- and T+ shifts to 8 while the dissociation to TO- and H+ shifts to 9. While the preference for dissociation into HO- and T+ agrees with experiment, the relative preference is not increasing with increasing temperature. This highlights that using simple scaling relationships for anharmonicities is not enough. Despite this, it is clear that the models suggest T+ rather than TO- as the right target.
II. Model for hydrogen isotope conduction through a narrow channel
Figure 1. Geometry of a Nafion channel based on average sulfonate side branch density and length. Less regular arrays would also be considered. |
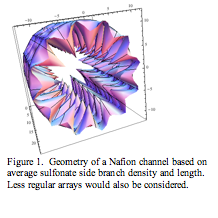
[1] L. R. Pratt, R. A. LaViolette, M. A. Gomez, and M. E. Gentile*, "Quasi-chemical Theory for the Statistical Thermodynamics of the Hard Sphere Fluid." J. Phys. Chem. B. 105, 11662 (2001); P. Grabowski*, D. Riccardi*, M. A. Gomez, D. Asthagiri, and L. R. Pratt, "Quasi-chemical theory and the standard free energy of H+ (aq)," J. Phys. Chem. A. 106, 9145 (2002); D. Asthagiri, L. R. Pratt, J. D. Kress, and M. A. Gomez, "The hydration state of HO-," Chemical Physics Letters 380, 530 (2003); D. Asthagiri, L. R. Pratt, J. D. Kress, and M. A. Gomez, "Hydration and mobility of HO- (aq)," Proc. Natl. Acad. Sci. USA 101, 7229 (2004).
[2] K. S. Schmidt-Rohr and Q. Chen, "Parallel cyllindrical water nanochannels in Nafion fuel-cell membranes," Nature Materials, 7, 75 (2008).
[3] T. S. Mahadevan and S. H. Garofallini, "Dissociative water potential for molecular dynamics simulations," J. Phys. Chem. B. 111, 8919 (2007).
[4] M. A. Gomez, and P. Peart*, "Including quantum subsystem character within classical equilibrium simulations," J. Chem. Phys. 125, 034105 (2006).
* denotes undergraduate collaborators